The Birch reduction is a chemical reaction named after the Australian chemist Arthur Birch. It is a method for the reduction of aromatic rings using alkali metals (usually sodium or lithium) in liquid ammonia. The reaction was first described by Arthur Birch in 1944.
In the Birch reduction, the aromatic ring undergoes a series of electron transfers and radical intermediates to eventually yield a substituted cyclohexadiene. The reaction is typically carried out at low temperatures (around -78°C) to maintain the liquid state of ammonia, which serves both as the solvent and the source of nitrogen radicals.
The general reaction scheme involves the addition of electrons and protons to the aromatic ring, leading to the formation of a radical anion intermediate. This intermediate can undergo further reactions, including protonation or addition of alkyl or alkoxy groups, depending on the specific reaction conditions and the presence of functional groups on the aromatic ring.
The Birch reduction is a powerful tool in organic synthesis for the selective reduction of aromatic compounds, particularly those containing electron-withdrawing substituents such as nitro, cyano, or carbonyl groups. It is widely used in the synthesis of various organic compounds, including natural products and pharmaceuticals. However, it should be noted that the reaction conditions must be carefully controlled to prevent over-reduction or side reactions.
The Birch reduction mechanism involves the stepwise addition of electrons and protons to an aromatic ring, resulting in the formation of a cyclohexadiene derivative. Here’s a simplified overview of the mechanism:
- Formation of Solvated Electrons: Alkali metals (typically sodium or lithium) are dissolved in liquid ammonia, producing solvated electrons (e^-_(solv)).
- Formation of Radical Anion: The solvated electrons (e^-_(solv)) attack the π-electron system of the aromatic ring, leading to the formation of a radical anion intermediate. This step is highly regioselective and occurs predominantly at positions ortho and para to electron-withdrawing substituents.
- Protonation: Protonation of the radical anion occurs, typically by ammonia itself, yielding a carbanion intermediate.
- Hydrogen Atom Transfer: A hydrogen atom is transferred from an ammonium ion (NH4^+) or an alkali metal-ammonia complex to the carbanion intermediate. This step generates a new radical intermediate.
- Second Protonation: The radical intermediate formed in step 4 is protonated again to yield a cyclohexadienyl radical.
- Formation of Cyclohexadiene: Two cyclohexadienyl radicals combine to form a cyclohexadiene derivative. This step is facilitated by the elimination of ammonia and the regeneration of solvated electrons, completing the reduction process.
The overall result of the Birch reduction is the conversion of an aromatic ring into a cyclohexadiene derivative with various substitution patterns, depending on the starting aromatic compound and reaction conditions. It’s worth noting that the Birch reduction is highly sensitive to reaction conditions, and controlling factors such as temperature and concentration is crucial for achieving the desired selectivity and efficiency in the reaction.
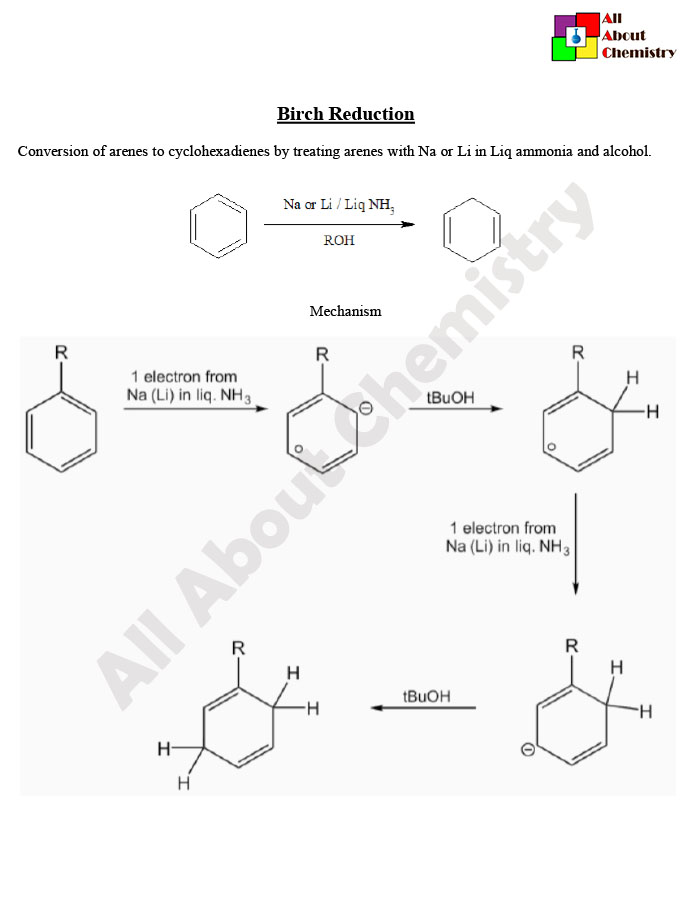
The Birch reduction is a versatile synthetic tool with several important applications in organic chemistry. Some notable applications include:
- Reduction of Aromatic Rings: The Birch reduction is primarily used for the selective reduction of aromatic rings, particularly those containing electron-withdrawing substituents such as nitro (-NO2), cyano (-CN), carbonyl (-CO), or halogen (-X) groups. This reaction allows for the conversion of aromatic compounds into cyclohexadiene derivatives, which are valuable intermediates in organic synthesis.
- Synthesis of Natural Products: The Birch reduction has been employed in the synthesis of various natural products and biologically active compounds. For example, it has been utilized in the synthesis of alkaloids, steroids, and terpenoids, among others. The ability to selectively reduce aromatic rings enables chemists to access complex molecular frameworks found in natural products.
- Functional Group Interconversions: The Birch reduction can be used to convert certain functional groups into others. For instance, aromatic nitro compounds can be reduced to primary amines, and aromatic carbonyl compounds can be converted to the corresponding alcohols. This provides a useful method for modifying functional groups within organic molecules.
- Total Synthesis of Pharmaceuticals: The Birch reduction has found applications in the total synthesis of pharmaceutical compounds. By selectively reducing specific aromatic rings, chemists can introduce key structural features required for the biological activity of target molecules. This has implications for the development of new drug candidates and the exploration of structure-activity relationships.
- Reduction of Aromatic Heterocycles: The Birch reduction can also be applied to aromatic heterocycles, such as pyridines and quinolines, leading to the formation of dihydropyridines and tetrahydroquinolines, respectively. These reduced heterocycles serve as important intermediates in the synthesis of various pharmaceuticals and agrochemicals.
Overall, the Birch reduction offers a powerful and selective method for modifying aromatic compounds, enabling the synthesis of diverse organic molecules with applications ranging from pharmaceuticals to materials science. However, it’s important to note that the reaction conditions must be carefully controlled to avoid over-reduction or side reactions.