Insecticides, pesticides, and chemical fertilizers are commonly used in agriculture to protect crops and increase yields. Learn about their uses and potential impacts here.
We will provide some interesting Chemistry Research topics , this will help you to gain more knowledge about different spheres of chemistry. CLICK
Insecticides
Insecticides are substances used to kill insects. They include ovicides and larvicides used against insect eggs and larvae, respectively. Insecticides are used in agriculture, medicine, industry and by consumers. Insecticides are claimed to be a major factor behind the increase in the 20th-century’s agricultural productivity. Nearly all insecticides have the potential to significantly alter ecosystems; many are toxic to humans and/or animals; some become concentrated as they spread along the food chain.

Insecticides can be classified into two major groups: systemic insecticides, which have residual or long term activity; and contact insecticides, which have no residual activity.
The mode of action describes how the pesticide kills or inactivates a pest. It provides another way of classifying insecticides. Mode of action can be important in understanding whether an insecticide will be toxic to unrelated species, such as fish, birds and mammals.
Insecticides may be repellent or non-repellent. Social insects such as ants cannot detect non-repellents and readily crawl through them. As they return to the nest they take insecticide with them and transfer it to their nestmates. Over time, this eliminates all of the ants including the queen. This is slower than some other methods, but usually completely eradicates the ant colony.
Types
Systemic insecticides
Systemic insecticides become incorporated and distributed systemically throughout the whole plant. When insects feed on the plant, they ingest the insecticide. Systemic insecticides produced by transgenic plants are called plant-incorporated protectants (PIPs). For instance, a gene that codes for a specific Bacillus thuringiensis biocidal protein was introduced into corn (maize) and other species. The plant manufactures the protein, which kills the insect when consumed.
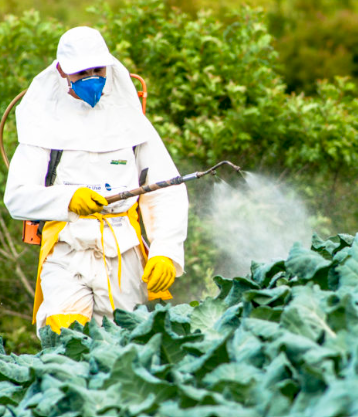
Contact insecticides
Contact insecticides are toxic to insects upon direct contact. These can be inorganic insecticides, which are metals and include the commonly used sulfur, and the less commonly used arsenates, copper and fluorine compounds. Contact insecticides can also be organic insecticides, i.e. organic chemical compounds, synthetically produced, and comprising the largest numbers of pesticides used today. Or they can be natural compounds like pyrethrum, neem oil, etc. Contact insecticides usually have no residual activity.
Efficacy can be related to the quality of pesticide application, with small droplets, such as aerosols often improving performance.
Synthetic insecticides
Organochlorides
The best known organochloride, DDT, was created by Swiss scientist Paul Müller. For this discovery, he was awarded the 1948 Nobel Prize for Physiology or Medicine. DDT was introduced in 1944. It functions by opening sodium channels in the insect’s nerve cells. The contemporaneous rise of the chemical industry facilitated large-scale production of DDT and related chlorinated hydrocarbons.
Organophosphates
Organophosphates are another large class of contact insecticides. These also target the insect’s nervous system. Organophosphates interfere with the enzymes acetylcholinesterase and other cholinesterases, disrupting nerve impulses and killing or disabling the insect. Organophosphate insecticides and chemical warfare nerve agents (such as sarin, tabun, soman, and VX) work in the same way. Organophosphates have a cumulative toxic effect to wildlife, so multiple exposures to the chemicals amplifies the toxicity. In the US, organophosphate use declined with the rise of substitutes.
Carbamates
Carbamate insecticides have similar mechanisms to organophosphates, but have a much shorter duration of action and are somewhat less toxic.
Pyrethroids
Pyrethroid pesticides mimic the insecticidal activity of the natural compound pyrethrin, the biopesticide found in Pyrethrum (Now Chrysanthemum and Tanacetum) species. These compounds are nonpersistent sodium channel modulators and are less toxic than organophosphates and carbamates. Compounds in this group are often applied against household pests.
Neonicotinoids
Neonicotinoids are synthetic analogues of the natural insecticide nicotine (with much lower acute mammalian toxicity and greater field persistence). These chemicals are acetylcholine receptor agonists. They are broad-spectrum systemic insecticides, with rapid action (minutes-hours). They are applied as sprays, drenches, seed and soil treatments. Treated insects exhibit leg tremors, rapid wing motion, stylet withdrawal (aphids), disoriented movement, paralysis and death. Imidacloprid may be the most common. It has recently come under scrutiny for allegedly pernicious effects on honeybees and its potential to increase the susceptibility of rice to planthopper attacks.
Phenylpyrazoles
Phenylpyrazole insecticides, such as fipronil are a class of synthetic insecticides that operate by interfering with GABA receptors.

Butenolides
Butenolide pesticides are a novel group of chemicals, similar to neonicotinoids in their mode of action, that have so far only one representative: flupyradifurone. They are acetylcholine receptor agonists, like neonicotinoids, but with a different pharmacophore. They are broad-spectrum systemic insecticides, applied as sprays, drenches, seed and soil treatments. Although the classic risk assessment considered this insecticide group (and flupyradifurone specifically) safe for bees, novel research has raised concern on their lethal and sublethal effects, alone or in combination with other chemicals or environmental factors.
Ryanoids/diamides
Diamides are synthetic ryanoid analogues with the same mode of action as ryanodine, a naturally occurring insecticide extracted from Ryania speciosa (Salicaceae). They bind to calcium channels in cardiac and skeletal muscle, blocking nerve transmission. The first insecticide from this class to be registered was Rynaxypyr, generic name chlorantraniliprole.
Detail Study
Insecticides are agents of chemical or biological origin that control insects. Control may result from killing the insect or otherwise preventing it from engaging in behaviors deemed destructive. Insecticides may be natural or manmade and are applied to target pests in a myriad of formulations and delivery systems (sprays, baits, slow-release diffusion, etc.). The science of biotechnology has, in recent years, even incorporated bacterial genes coding for insecticidal proteins into various crop plants that deal death to unsuspecting pests that feed on them.
The purpose of this brief chapter is to provide a handshake overview of what insecticides are, and a short background and a review of the major insecticide classes that have been or are used today to cope with insect pests. Though by no means exhaustive, we will touch on major classes and technologies whether decades old or recently revealed.
Some 10,000 species of the more than 1 million species of insects are crop-eating, and of these, approximately 700 species worldwide cause most of the insect damage to man’s crops, in the field and in storage.
Humanoids have been on earth for more than 3 million years, while insects have existed for at least 250 million years. We can guess that among the first approaches used by our primitive ancestors to reduce insect annoyance was hugging smoky fires or spreading mud and dust over their skin to repel biting and tickling insects, a practice resembling the habits of elephants, swine, and water buffalo. Today, such approaches would be classed as repellents, a category of insecticides.
Historians have traced the use of pesticides to the time of Homer around 1000 B.C., but the earliest records of insecticides pertain to the burning of “brimstone” (sulfur) as a fumigant. Pliny the Elder (A.D. 23-79) recorded most of the earlier insecticide uses in his Natural History. Included among these were the use of gall from a green lizard to protect apples from worms and rot. Later, we find a variety of materials used with questionable results: extracts of pepper and tobacco, soapy water, whitewash, vinegar, turpentine, fish oil, brine, lye among many others.
At the beginning of World War II (1940), our insecticide selection was limited to several arsenicals, petroleum oils, nicotine, pyrethrum, rotenone, sulfur, hydrogen cyanide gas, and cryolite. It was World War II that opened the Modern Era of Chemical control with the introduction of a new concept of insect control –synthetic organic insecticides, the first of which was DDT.
Organochlorines
The organochlorines are insecticides that contain carbon (thus organo-), hydrogen, and chlorine. They are also known by other names: chlorinated hydrocarbons, chlorinated organics, chlorinated insecticides, and chlorinated synthetics. The organochlorines are now primarily of historic interest, since few survive in today’s arsenal.
Diphenyl Aliphatics
The oldest group of the organochlorines is the diphenyl aliphatics, which included DDT, DDD, dicofol, ethylan, chlorobenzilate, and methoxychlor. DDT is probably the best known and most notorious chemical of the 20th century. It is also fascinating, and remains to be acknowledged as the most useful insecticide developed. More than 4 billion pounds of DDT were used throughout the world, beginning in 1940, and in the U.S. ending essentially in 1973, when the U.S. Environmental Protection Agency canceled all uses. The remaining First World countries rapidly followed suit. DDT is still effectively used for malaria control in several third world countries. In 1948, Dr. Paul Muller, a Swiss entomologist, was awarded the Nobel Prize in Medicine for his lifesaving discovery of DDT (1939) as an insecticide useful in the control of malaria, yellow fever and many other insect-vectored diseases.
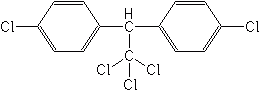
Mode of action
The mode of action for DDT has never been clearly established, but in some complex manner it destroys the delicate balance of sodium and potassium ions within the axons of the neuron in a way that prevents normal transmission of nerve impulses, both in insects and mammals. It apparently acts on the sodium channel to cause “leakage” of sodium ions. Eventually the affected neurons fire impulses spontaneously, causing the muscles to twitch– “DDT jitters”– followed by convulsions and death. DDT has a negative temperature correlation–the lower the surrounding temperature the more toxic it becomes to insects.
Hexchlorocyclohexane (HCH)
Also known as benzenehexachloride (BHC), the insecticidal properties of HCH were discovered in 1940 by French and British entomologists. In its technical grade, there are five isomers, alpha, beta, gamma, delta and epsilon. Surprisingly, only the gamma isomer has insecticidal properties. Consequently, the gamma isomer was isolated in manufacture and sold as the odorless insecticide lindane. In contrast, technical grade HCH has a strong musty odor and flavor, which can be imparted to treated crops and animal products. Because of its very low cost, HCH is still used in many developing countries. In 2002, the U.S. EPA removed all food-related (tolerance-requiring) uses of lindane from the U.S.
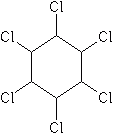
Mode of action
The effects of HCH superficially resemble those of DDT, but occur much more rapidly, and result in a much higher rate of respiration in insects. The gamma isomer is a neurotoxicant whose effects are normally seen within hours as increased activity, tremors, and convulsions leading to prostration. It too, exhibits a negative temperature correlation, but not as pronounced as that of DDT.
Cyclodienes
The cyclodienes appeared after World War II: chlordane, 1945, aldrin and dieldrin, 1948; heptachlor, 1949; endrin, 1951; mirex, 1954; endosulfan, 1956; and chlordecone (Kepone®), 1958. There were other cyclodienes of minor importance developed in the U.S. and Germany. Most of the cyclodienes are persistent insecticides and are stable in soil and relatively stable to the ultraviolet of sunlight. As a result, they were used in greatest quantity as soil insecticides (especially chlordane, heptachlor, aldrin, and dieldrin) for the control of termites and soil-borne insects whose larval stages feed on the roots of plants.
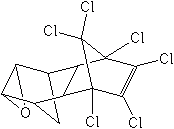
To appreciate the effectiveness of these materials as termiticides, consider that wood and wooden structures treated with chlordane, aldrin, and dieldrin in the year of their development are still protected from damage—after more than 60 years! The cyclodienes were the most effective, long-lasting and economical termiticides ever developed. Because of their persistence in the environment, resistance that developed in several soil insect pests, and in some instances biomagnification in wildlife food chains, most agricultural uses of cyclodienes were canceled by the EPA between 1975 and 1980, and their use as termiticides canceled in 1984-88.
Mode of action
Unlike DDT and HCH, the cyclodienes have a positive temperature correlation–their toxicity increases with increasing ambient temperature. Their modes of action are also not clearly understood. However, it is known that this group acts on the inhibitory mechanism called the GABA (g-aminobutyric acid) receptor. This receptor operates by increasing chloride ion permeability of neurons. Cyclodienes prevent chloride ions from entering the neurons, and thereby antagonize the “calming” effects of GABA. Cyclodienes appear to affect all animals similarly, first with the nervous activity followed by tremors, convulsions and prostration.
Polychloroterpenes
Only two polychloroterpenes were developed–toxaphene in 1947, and strobane in 1951. Toxaphene had by far the greatest use of any single insecticide in agriculture, while strobane was relatively insignificant. Toxaphene was used on cotton, first in combination with DDT, for alone it had minimal insecticidal qualities. Then, in 1965, after several major cotton insects became resistant to DDT, toxaphene was formulated with methyl parathion, an organophosphate insecticide mentioned later.
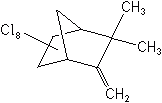
Toxaphene is a mixture of more than 177 10-carbon polychlorinated derivatives. These materials persist in the soil, though not as long as the cyclodienes, and disappear from the surfaces of plants in 3-4 weeks. This disappearance is attributed more to volatility than to photolysis or plant metabolism. Toxaphene is rather easily metabolized by mammals and birds, and is not stored in body fat nearly to the extent of DDT, HCH and the cyclodienes. Despite its low toxicity to insects, mammals and birds, fish are highly susceptible to toxaphene poisoning, in the same order of magnitude as to the cyclodienes. Toxaphene’s registrations were canceled by EPA in 1983.
Mode of action
Toxaphene and strobane act on the neurons, causing an imbalance in sodium and potassium ions, similar to that of the cyclodiene insecticides.
Organophosphates
Organophosphates (OPs) is the term that includes all insecticides containing phosphorus. Other names used, but no longer in vogue, are organic phosphates, phosphorus insecticides, nerve gas relatives and phosphoric acid esters. All organophosphates are derived from one of the phosphorus acids, and as a class are generally the most toxic of all pesticides to vertebrates. Because of the similarity of OP chemical structures to the “nerve gases,” their modes of action are also similar. Their insecticidal qualities were first observed in Germany during World War II in the study of the extremely toxic OP nerve gases sarin, soman, and tabun. Initially, the discovery was made in search of substitutes for nicotine, which was heavily used as an insecticide but in short supply in Germany.
The OPs have two distinctive features: they are generally more toxic to vertebrates than other classes of insecticides, and most are chemically unstable or nonpersistent. It is this latter characteristic that brought them into agricultural use as substitutes for the persistent organochorines. Because of the relatively high toxicity of the OP’s, EPA, under provisions of the Food Quality Protection Act (1996), undertook an extensive reappraisal of the entire class beginning in the late 1990’s. Many OP’s were voluntarily canceled and others lost uses.
Mode of action
The OPs work by inhibiting certain important enzymes of the nervous system, namely cholinesterase (ChE). The enzyme is said to be phosphorylated when it becomes attached to the phosphorous moiety of the insecticide, a binding that is irreversible. This inhibition results in the accumulation of acetylcholine (ACh) at the neuron/neuron and neuron/muscle (neuromuscular) junctions or synapses, causing rapid twitching of voluntary muscles and finally paralysis.
Classification
All OPs are esters of phosphorus having varying combinations of oxygen, carbon, sulfur and nitrogen attached, resulting in six different subclasses: phosphates, phospho-nates, phosphorothioates, phosphorodithioates, phosphorothiolates and phosphoramidates. These subclasses are easily identified by their chemical names.
The OPs are generally divided into three groups–aliphatic, phenyl, and heterocyclic derivatives.
Aliphatics
The aliphatic OPs are carbon chain-like in structure. The first OP brought to agriculture, TEPP (1946) belonged to this group. Other examples are malathion, trichlorfon (Dylox®), monocrotophos (Azodrin®), dimethoate (Cygon®), oxydemetonmethyl (Meta Systox®), dicrotophos (Bidrin®), disulfoton (Di-Syston®), dichlorvos (Vapona®), mevinphos (Phosdrin®), methamidophos (Monitor®), and acephate (Orthene®).
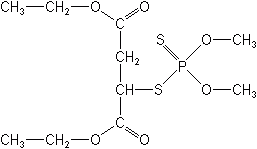
Phenyl derivatives
The phenyl OPs contain a phenyl ring with one of the ring hydrogens displaced by attachment to the phosphorus moiety and other hydrogens frequently displaced by Cl, NO2, CH3, CN, or S. The phenyl OPs are generally more stable than the aliphatics, thus their residues are longer lasting. The first phenyl OP brought into agriculture was parathion (ethyl parathion) in 1947. Examples of other phenyl OPs are methyl parathion, profenofos (Curacron®), sulprofos (Bolstar®), isofenphos (Oftanol®, Pryfon®), fenitrothion (Sumithion®), fenthion (Dasanit®), and famphur (Cyflee®. Warbex®).
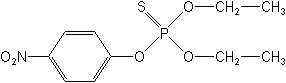
Heterocyclic derivatives
The term heterocyclic means that the ring structures are composed of different or unlike atoms, e.g.,oxygen, nitrogen or sulfur. The first of this group was diazinon introduced in 1952. Other examples in this group are azinphos-methyl (Guthion®), azinphos-ethyl (Acifon®, Gusathion®), chlorpyrifos (Dursban®, Lorsban®, Lock-On®), methidathion (Supracide®), phosmet (Imidan®), isazophos (Brace®, Triumph®), and chlorpyrifos-methyl (Reldan®).
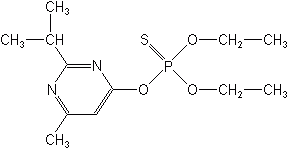
Organosulfurs
These few materials have very low toxicity to insects and are used only as acaricides (miticides). They contain two phenyl rings, resembling DDT, with sulfur in place of carbon as the central atom. These include tetradifon (Tedion®), propargite (Omite®, Comite®), and ovex (Ovotran®).
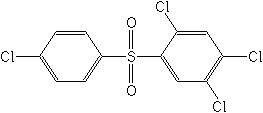
Carbamates
The carbamate insecticides are derivatives of carbamic acid (as the OPs are derivatives of phosphoric acid). And like the OPs, their mode of action is that of inhibiting the vital enzyme cholinesterase (ChE).
The first successful carbamate insecticide, carbaryl (Sevin®), was introduced in 1956. More of it has been used worldwide than all the remaining carbamates combined. Two distinct qualities have made it the most popular carbamate: its very low mammalian oral and dermal toxicity and an exceptionally broad spectrum of insect control. Other long-standing carbamate insecticides are methomyl (Lannate®), carbofuran (Furadan®), aldicarb (Temik®), oxamyl (Vydate®), thiodicarb (Larvin®), methiocarb (Mesurol®), propoxur (Baygon®), bendiocarb (Ficam®), carbosulfan (Advantage®), aldoxycarb (Standak®), promecarb (Carbamult®), and fenoxycarb (Logic®, Torus®). Carbamates more recently introduced include primicarb, indoxacarb (registered in 2000), alanycarb and furathiocarb.
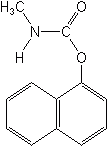
Mode of action
Carbamates inhibit cholinesterase (ChE) as OPs do, and they behave in almost identical manner in biological systems, but with two main differences. Some carbamates are potent inhibitors of aliesterase (miscellaneous aliphatic esterases whose exact functions are not known), and their selectivity is sometimes more pronounced against the ChE of different species. Second, ChE inhibition by carbamates is reversible. When ChE is inhibited by a carbamate, it is said to be carbamylated, as when an OP results in the enzyme being phosphorylated. In insects, the effects of OPs and carbamates are primarily those of poisoning of the central nervous system, since the insect neuromuscular junction is not cholinergic, as in mammals. The only cholinergic synapses known in insects are in the central nervous system. (The chemical neuromuscular junction transmitter in insects is thought to be glutamic acid.)
Formamidines
The formamidines comprise a small group of insecticides. Three examples are chlordimeform (Galecron®, Fundal®), which is no longer registered in the U.S., formetanate (Carzol®), and amitraz (Mitac®, Ovasyn®). Their current value lies in the control of OP- and carbamate-resistant pests.
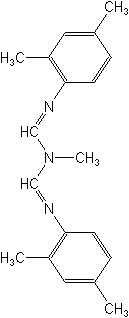
Mode of action
Formamidine poisoning symptoms are distinctly different from other insecticides. Their proposed action is the inhibition of the enzyme monoamine oxidase, which is responsible for degrading the neurotransmitters norepinephrine and serotonin. This results in the accumulation of these compounds, which are known as biogenic amines. Affected insects become quiescent and die.
Dinitrophenols
The basic dinitrophenol molecule has a broad range of toxicities–as herbicides, insecticides, ovicides, and fungicides. Of the insecticides, binapacryl (Morocide®) and dinocap (Karathane®) were the most recently used. Dinocap is an effective miticide and was very heavily used as a fungicide for the control of powdery mildew fungi. Because of the inherent toxicity of the dinitrophenols, they have all been withdrawn.
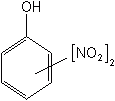
Mode of action
Dinitrophenols act by uncoupling or inhibiting oxidative phosphorylation, which basically prevents the formation of the high-energy phosphate molecule, adenosine triphosphate (ATP).
Organotins
The organotins are a group of acaricides that double as fungicides. Of particular interest is cyhexatin (Plictran®), one of the most selective acaricides known, introduced in 1967. Fenbutatin-oxide (Vendex®) has been used extensively against mites on deciduous fruits, citrus, greenhouse crops, and ornamentals.
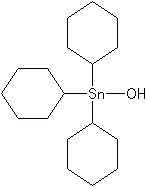
Mode of action
These tin compounds inhibit oxidative phosphorylation at the site of dinitrophenol uncoupling, preventing the formation of the high-energy phosphate molecule adenosine triphosphate (ATP). These trialkyl tins also inhibit photophosphorylation in chloroplasts, the chlorophyll-bearing subcellular units) and could therefore serve as algicides.
Pyrethroids
Natural pyrethrum has seldom been used for agricultural purposes because of its cost and instability in sunlight. In recent decades, many synthetic pyrethrin-like materials have become available. They were originally referred to as synthetic pyrethroids. Currently, the better nomenclature is simply pyrethroids. These are stable in sunlight and are generally effective against most agricultural insect pests when used at the very low rates of 0.01 to 0.1 pound per acre.
The pyrethroids have an interesting evolution, which is conveniently divided into four generations. The first generation contains only one pyrethroid, allethrin (Pynamin®), which appeared in 1949. Its synthesis was very complex, involving 22 chemical reactions to reach the final product.
The second generation includes tetramethrin (Neo-Pynamin®) (1965), followed by resmethrin (Synthrin®) in 1967 (20X as effective as pyrethrum), then bioresmethrin (50X as effective as pyrethrum) (1967), then Bioallethrin® (1969), and finally phonothrin (Sumithrin®) (1973).
The third generation includes fenvalerate (Pydrin®[discontinued], Tribute®, & Bellmark®), and permethrin (Ambush®, Astro®, Dragnet®, Flee®, Pounce®, Prelude®, Talcord®& Torpedo®) which appeared in 1972-73. These became the first agricultural pyrethroids because of their exceptional insecticidal activity (0.1 lb ai/A) and their photostability. They were virtually unaffected by ultraviolet in sunlight, lasting 4-7 days as efficacious residues on crop foliage.
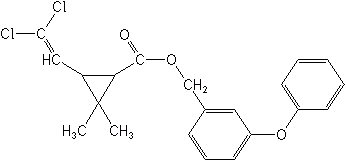
The fourth and current generation, is truly exciting because of their effectiveness in the range of 0.01 to 0.05 lb ai/A. These include bifenthrin (Capture®, Talstar®), lambda-cyhalothrin (Demand®, Karate®, Scimitar®& Warrior®), cypermethrin (Ammo®, Barricade®, Cymbush®, Cynoff® & Ripcord®), cyfluthrin (Baythroid®, Countdown®, Cylense®, Laser® & Tempo®), deltamethrin (Decis®) esfenvalerate (Asana®, Hallmark®), fenpropathrin (Danitol®), flucythrinate (Cybolt®, Payoff®), fluvalinate (Mavrik®, Spur ®, discontinued), prallethrin (Etoc®), tau-fluvalinate (Mavrik®) tefluthrin (Evict®, Fireban®, Force® & Raze®), tralomethrin (Scout X-TRA®, Tralex®), and zeta-cypermethrin (Mustang® & Fury®). All of these are photostable, that is, they do not undergo photolysis (splitting) in sunlight. And because they have minimal volatility they provide extended residual effectiveness, up to 10 days under optimum conditions.
Recent additions to the fourthgeneration pyrethroids are acrinathrin (Rufast®), imiprothrin (Pralle®), registered in 1998, and gamma-cyhalothrim (Pytech®), which is in development.
Mode of action
The pyrethroids share similar modes of action, resembling that of DDT, and are considered axonic poisons. They apparently work by keeping open the sodium channels in neuronal membranes. There are two types of pyrethroids. Type I, among other physiological responses, have a negative temperature coefficient, resembling that of DDT. Type II, in contrast have a positive temperature coefficient, showing increased kill with increase in ambient temperature. Pyrethroids affect both the peripheral and central nervous system of the insect. They initially stimulate nerve cells to produce repetitive discharges and eventually cause paralysis. Such effects are caused by their action on the sodium channel, a tiny hole through which sodium ions are permitted to enter the axon to cause excitation. The stimulating effect of pyrethroids is much more pronounced than that of DDT.
Nicotinoids
The nicotinoids are a newer class of insecticides with a new mode of action. They have been previously referred to as nitro-quanidines, neonicotinyls, neonicotinoids, chloronicotines, and more recently as the chloronicotinyls. Just as the synthetic pyrethroids are similar to and modeled after the natural pyrethrins, so too, are the nicotinoids similar to and modeled after the natural nicotine . Imidacloprid was introduced in Europe and Japan in 1990 and first registered in the U.S. in 1992. It is currently marketed as several proprietary products worldwide, e.g., Admire®, Confidor®, Gaucho®, Merit®, Premier®, Premise® and Provado®. Very possibly it is used in the greatest volume globally of all insecticides.
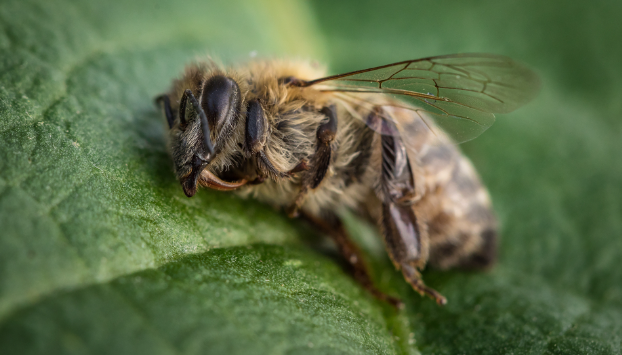
Imidacloprid is a systemic insecticide, having good root-systemic characteristics and notable contact and stomach action. It is used as a soil, seed or foliar treatment in cotton, rice cereals, peanuts, potatoes, vegetables, pome fruits, pecans and turf, for the control of sucking insects, soil insects, whiteflies, termites, turf insects and the Colorado potato beetle, with long residual control. Imidacloprid has no effect on mites or nematodes.
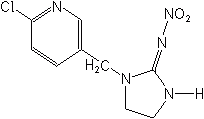
Other nicotinoids include acetamiprid (Assail®), thiamethoxam (Actara®, Platinum®), nitenpyram (Bestguard®), clothianidin (Poncho®, dinotefuran (Starke®) and thiacloprid. U.S. registrations for acetamiprid, thiamethoxam and thiacloprid were granted in 2002 and clothianidin in 2003.
Mode of action
The nicotinoids act on the central nervous system of insects, causing irreversible blockage of postsynaptic nicotinergic acetylcholine receptors (See also Nicotine under the Botanicals).
Spinosyns
Spinosyns are among the newest classes of insecticides, represented by spinosad (Success®, Tracer Naturalyte®). Spinosad is a fermentation metabolite of the actinomycete Saccharopolyspora spinosa, a soil-inhabiting microorganism. It has a novel molecular structure and mode of action that provide excellent crop protection typically associated with synthetic insecticides, first registered for use on cotton in 1997. Spinosad is a mixture of spinosyns A and D (thus its name, spinosAD). It is particularly effective as a broad-spectrum material for most caterpillar pests at the astonishing rates of 0.04 to 0.09 pound of active ingredient (18 to 40 grams) per acre. It has both contact and stomach activity against lepidopteran larvae, leaf miners, thrips, and termites, with long residual activity. Crops registered include cotton, vegetables, tree fruits, ornamentals and others.

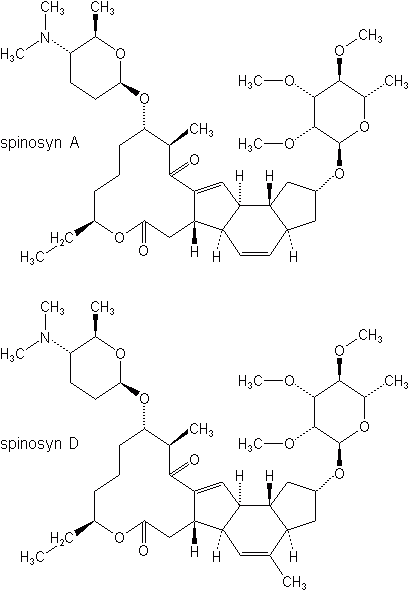
Mode of action
Spinosad acts by disrupting binding of acetylcholine in nicotinic acetylcholine receptors at the postsynaptic cell (Salgado VL 1997) (See also Nicotine under the Botanicals).
Fiproles (or Phenylpyrazoles)
Fipronil (Regent®, Icon®, Frontline®) is the only insecticide in this new class, introduced in 1990 and registered in the U.S. in 1996. It is a systemic material with contact and stomach activity. Fipronil is used for the control of many soil and foliar insects, (e.g., corn rootworm, Colorado potato beetle, and rice water weevil) on a variety of crops, primarily corn, turf, and for public health insect control. It is also used for seed treatment and formulated as baits for cockroaches, ants and termites. Fipronil is effective against insects resistant or tolerant to pyrethroid, organophosphate and carbamate insecticides.
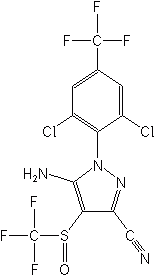
Mode of action
Fipronil blocks the (g-aminobutyric acid- (GABA) regulated chloride channel in neurons, thus antagonizing the “calming” effects of GABA, similar to the action of the Cyclodienes (see page 3).
Pyrroles
Chlorfenapyr (Alert®, Pirate®) is the first and only member of this unique chemical group, as both a contact and stomach insecticide-miticide. It is used on cotton and experimentally on corn, soybeans, vegetables, tree and vine crops, and ornamentals to control whitefly, thrips, caterpillars, mites, leafminers, aphids, and Colorado potato beetle. It has ovicidal activity on some species. EPA took the unusual step of refusing to register chlorfenapyr in 2000 for cotton insect control because of potential hazards to birds. However, labels for greenhouse ornamentals were granted in 2001.
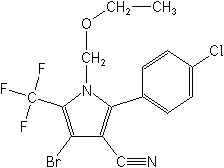
Mode of action
Chlorfenapyr is an “uncoupler” or inhibitor of oxidative phosphorylation, preventing the formation of the crucial energy molecule adenosine triphosphate (ATP) (See also Dinitrophenols).
Pyrazoles
The original pyrazoles were tebufenpyrad and fenpyroximate (not illustrated). These were designed primarily as non-systemic contact and stomach miticides, but do have limited effectiveness on psylla, aphids, whitefly, and thrips. Tebufenpyrad (Pyranica®, Masai®), registered by EPA in 2002, is used on cotton, soybeans, vegetables, pome fruits, grapes and citrus. Fenpyroximate (Acaban®, Dynamite®) controls all stages of mites, gives fast knockdown, inhibits molting of immature stages of mites, and has long residual activity. Newer members of this class include ethiprole (Curbex®) which is active on a broad sprectum of chewing and sucking insects, and tolfenpyrad (OMI-88) which is reputed to active on pests infesting cole and cucurbit crops.
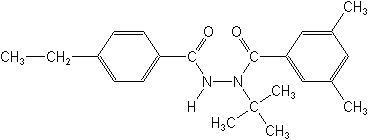
Mode of action
Their mode of action is that of inhibiting mitochondrial electron transport at the NADH-CoQ reductase site, leading to the disruption of adenosine triphosphate (ATP) formation, the crucial energy molecule.
Pyridazinones
Pyridaben (Nexter®, Sanmite®) is the only member of this class. It is a selective contact insecticide and miticide, also effective against thrips, aphids, whiteflies and leafhopprs. Registrations are for pome fruits, almonds, citrus, ornamentals and greenhouse ornamentals. Pyridaben provides exceptionally long residual control, and rapid knockdown at a broad range of temperatures.
Mode of action
Pyridaben is a metabolic inhibitor that interrupts mitochondrial electron transport at Site 1, similar to the Quinazolines, below.
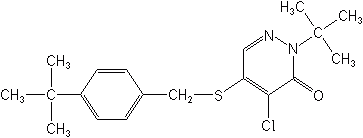
Quinazolines
The quinazolines offer a unique chemical configuration, consisting only of one insecticide, fenazaquin (Matador®). Fenazaquin is a contact and stomach miticide. It has ovicidal activity, gives rapid knockdown, and controls all stages of mites. Not yet registered in the U.S., it is used on cotton, stone and pome fruits, citrus, grapes and ornamentals.
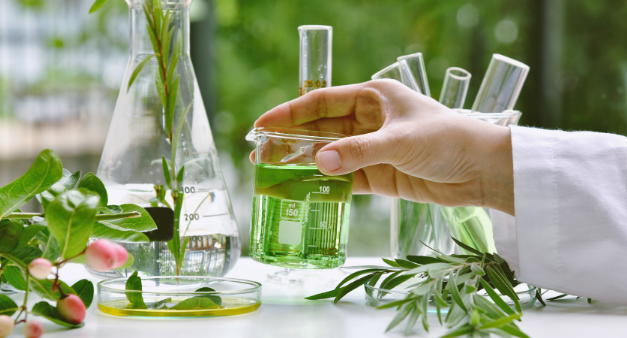
FENAZAQUIN (Matador®)
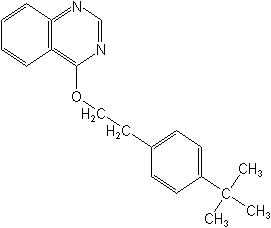
Mode of action
Fenazaquin inhibits mitochondrial electron transport at Site 1, similar to the Pyridazinones, above.
Benzoylureas
Benzoylureas are an entirely different class of insecticides that act as insect growth regulators (IGRs). Rather than being the typical poisons that attack the insect nervous system, they interfere with chitin synthesis and are taken up more by ingestion than by contact. Their greatest value is in the control of caterpillars and beetle larvae.
Benzoylureas were first used in Central America in 1985, to control a severe, resistant leafworm complex (Spodoptera spp., Trichoplusia spp.) outbreak in cotton. The withdrawal of the ovicide chlordimeform made their control quite difficult due to their high resistance to almost all insecticide classes, including the pyrethroids.
The benzoylureas were introduced in 1978 by Bayer of Germany, triflumuron (Alsystin®) being the first. Others appearing since then are chlorfluazuron (Atabron®), followed by teflubenzuron (Nomolt®, Dart®), hexaflumuron (Trueno®, Consult®), flufenoxuron (Cascade®), and flucycloxuron (Andalin®). Others are flurazuron, novaluron, and diafenthiuron, bistrifluron (DBI-3204) and noviflumuron (XDE-007). Until recently lufenuron (Axor®) was the newest addition to this group, appearing in 1990. Among the newer benzoylureas only hexaflumuron (1993) and novaluron (2001) have been registered by EPA.
The only other benzoylurea registered in the U.S. is diflubenzuron (Dimilin®, Adept®, Micromite®). It was first registered in 1982 for gypsy moth, cotton boll weevil, most forest caterpillars, soybean caterpillars, and mushroom flies, but now with a much broader range of registrations.
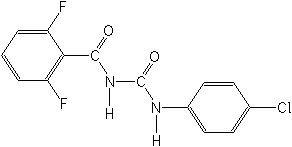
Though not a benzoylurea, cyromazine (Larvadex®, Trigard®), a triazine, is also a potent chitin synthesis inhibitor. It is selective toward Dipterous species and used for the control of leafminers in vegetable crops and ornamentals, and fed to poultry or sprayed to control flies in manure of broiler and egg producing operations, and incorporated into compost of mushroom houses for fungus gnats.
Mode of action
The benzoylureas act on the larval stages of most insects by inhibiting or blocking the synthesis of chitin, a vital and almost indestructible part of the insect exoskeleton. Typical effects on developing larvae are the rupture of malformed cuticle or death by starvation. Adult female boll weevils exposed to diflubenzuron lay eggs that do not hatch. And, mosquito larvae control can be achieved with as little as 1.0 gram of diflubenzuron per acre of surface water.
Botanicals
Botanical insecticides are of great interest to many, for they are natural insecticides, toxicants derived from plants. Historically, the plant materials have been in use longer than any other group, with the possible exception of sulfur. Tobacco, pyrethrum, derris, hellebore, quassia, camphor, and turpentine were some of the more important plant products in use before the organized search for insecticides began in the early 1940s.
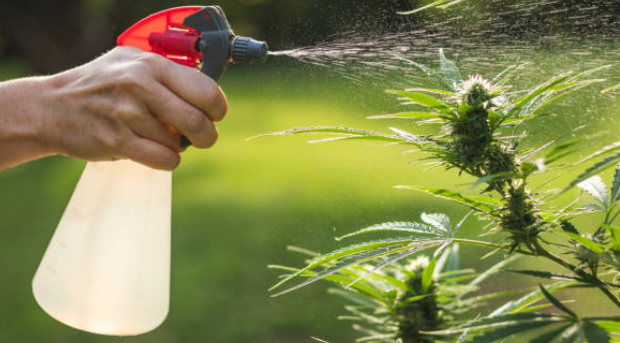
In recent years the term biorational has been put into play by the EPA. There are similarities and differences between the terms botanical and biorational. We include a section on the biorationals below and will address the overlaps in that section.
Botanical insecticide use in the U.S. peaked in 1966, and has declined steadily since. Pyrethrum is now the only classical botanical of significance in use. Some newer plant-derived insecticides that have come into use are referred to as florals or scented plant chemicals and include, among others, limonene, cinnamaldehyde and eugenol. In addition, there is azadirachtin from the neem tree which is used in greenhouse and on ornamentals.
Pyrethrum
Pyrethrum is extracted from the flowers of a chrysanthemum grown in Kenya and Ecuador. It is one of the oldest and safest insecticides available. The ground, dried flowers were used in the early 19th century as the original louse powder to control body lice in the Napoleonic Wars. Pyrethrum acts on insects with phenomenal speed causing immediate paralysis, thus its popularity in fast knockdown household aerosols. However, unless it is formulated with one of th synergists, most of the paralyzed insects recover to once again become pests. Pyrethrum is a mixture of four compounds: pyrethrins I and II and cinerins I and II.

Mode of action
Pyrethrum is an axonic poison, as are the synthetic pyrethroids and DDT. Axonic poisons are those that in some way affect the electrical impulse transmission along the axons, the elongated extensions of the neuron cell body. Pyrethrum and some pyrethroids have a greater insecticidal effect when the temperature is lowered, a negative temperature coefficient, as does DDT. They affect both the peripheral and central nervous system of the insect. Pyrethrum initially stimulates nerve cells to produce repetitive discharges, leading eventually to paralysis. Such effects are caused by their action on the sodium channel, a tiny hole through which sodium ions are permitted to enter the axon to cause excitation. These effects are produced in insect nerve cord, which contains ganglia and synapses, as well as in giant nerve fiber axons.
Nicotine
Nicotine is extracted by several methods from tobacco, and is effective against most all types of insect pests, but is used particularly for aphids and caterpillars–soft bodied insects. Nicotine is an alkaloid, a chemical class of heterocyclic compounds containing nitrogen and having prominent physiological properties. Other well-known alkaloids that are not insecticides are caffeine (coffee, tea), quinine (cinchona bark), morphine (opium poppy), cocaine (coca leaves), ricinine (a poison in castor oil beans), strychnine (Strychnos nux vomica), coniine (spotted hemlock, the poison used by Socrates), and, finally LSD (a hallucinogen from the ergot fungus attacking grain).

Mode of action
Nicotine action is one of the first, classic modes of action identified by pharmacologists. Drugs that act similarly to nicotine are said to have a nicotinic response. Nicotine mimics acetylcholine (ACh) at the neuromuscular (nerve/muscle) junction in mammals, and results in twitching, convulsions, and death, all in rapid order. In insects the same action is observed, but only in the central nervous system ganglia.
Rotenone
Rotenone or rotenoids are produced in the roots of two genera of the legume family: Derris and Lonchocarpus (also called cubé) grown in South America. It is both a stomach and contact insecticide and used for the last century and a half to control leaf-eating caterpillars, and three centuries prior to that in South America to paralyze fish, causing them to surface and be easily captured. Today, rotenone is used in the same way to reclaim lakes for game fishing. Used on a prescribed basis, it eliminates all fish, closing the lake to reintroduction of rough species. It is a selective piscicide in that it kills all fish at dosages that are relatively nontoxic to fish food organisms, and is degraded rapidly.
Mode of action
Rotenone is a respiratory enzyme inhibitor, acting between NAD+ (a coenzyme involved in oxidation and reduction in metabolic pathways) and coenzyme Q (a respiratory enzyme responsible for carrying electrons in some electron transport chains), resulting in failure of the respiratory functions.
Limonene or d-Limonene
Limonene or d-Limonene is the latest addition to the botanicals. Limonene belongs to a group often called florals or scented plant chemicals. Extracted from citrus peel, it is effective against all external pests of pets, including fleas, lice, mites, and ticks, and is virtually nontoxic to warm-blooded animals. Several insecticidal substances occur in citrus oil, but the most important is limonene, which constitutes about 98% of the orange peel oil by weight. Two other recently introduced floral products are eugenol (Oil of Cloves) and cinnamaldehyde (derived from Ceylon and Chinese cinnamon oils). They are used on ornamentals and many crops to control various insects.
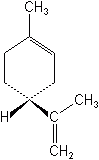
Mode of action
Its mode of action is similar to that of pyrethrum. It affects the sensory nerves of the peripheral nervous system, but it is not a ChE inhibitor.
Neem
Neem oil extracts are squeezed from the seeds of the neem tree and contain the active ingredient azadirachtin, a nortriterpenoid belonging to the lemonoids. Azadirachtin has shown some rather sensational insecticidal, fungicidal and bactericidal properties, including insect growth regulating qualities. Azatin® is marketed as an insect growth regulator, and Align® and Nemix® as a stomach/contact insecticide for greenhouse and ornamentals.

Mode of action
Azadirachtin disrupts molting by inhibiting biosynthesis or metabolism of ecdysone, the juvenile molting hormone.
Synergists or Activators
Synergists are not in themselves considered toxic or insecticidal, but are materials used with insecticides to synergize or enhance the activity of the insecticides. The first was introduced in 1940 to increase the effectiveness of pyrethrum. Since then many materials have appeared, but only a few are still marketed. Synergists are found in most all household, livestock and pet aerosols to enhance the action of the fast knockdown insecticides pyrethrum, allethrin, and resmethrin, against flying insects. Current synergists, such as piperonyl butoxide, contain the methylenedioxyphenyl moiety, a molecule found in sesame oil and later named sesamin.
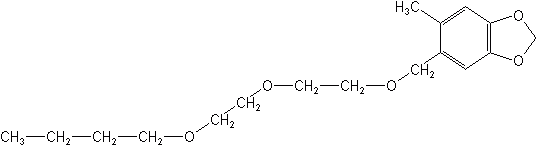
Mode of action
The synergists inhibit cytochrome P-450 dependent polysubstrate monooxygenases (PSMOs), enzymes produced by microsomes, the subcellular units found in the liver of mammals and in some insect tissues ( e.g., fat bodies). The earlier name for these enzymes was mixed-function oxidases (MFOs). These PSMOs bind the enzymes that degrade selected foreign substances, such as pyrethrum, allethrin, resmethrin or any other synergized compound. Synergists simply bind the oxidative enzymes and prevent them from degrading the toxicant.
Antibiotics
In this category belong the avermectins, which are insecticidal, acaricidal, and antihelminthic agents that have been isolated from the fermentation products of Streptomyces avermitilis, a member of the actinomycete family. Abamectin is the common name assigned to the avermectins, a mixture of containing 80% avermectin B1a and 20% B1b, homologs that have about equal biological activity. Clinch® is a fire ant bait, and Avid® is applied as a miticide/insecticide. Abamectin has certain local systemic qualities, permitting it to kill mites on a leaf’s underside when only the upper surface is treated. The most promising uses for these materials are the control of spider mites, leafminers and other difficult-to-control greenhouse pests, and internal parasites of domestic animals.
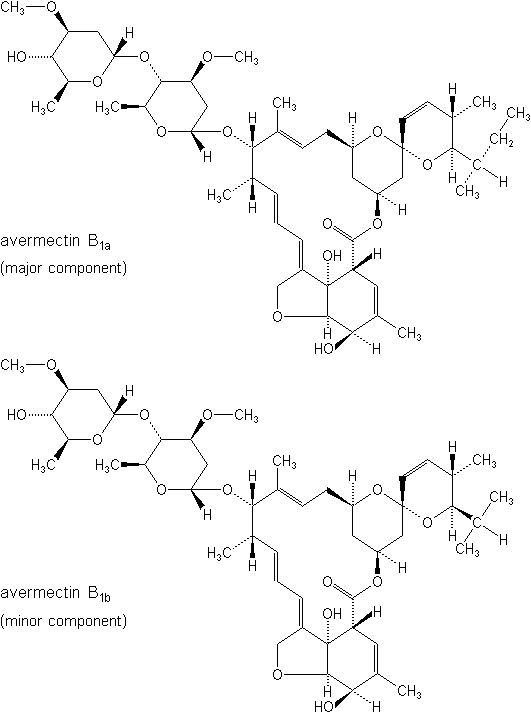
Emamectin benzoate (Proclaim®, Denim®) is an analog of abamectin, produced by the same fermentation system as abamectin. It was first registered in 1999. It is both a stomach and contact insecticide used primarily for control of caterpillars at the rate of 0.0075 to 0.015 lb (3.5 to 7.0 grams) a.i. per acre. Shortly after exposure, larvae stop feeding and become irreversibly paralyzed, dying in 3-4 days. Rapid photodegradation of both abamectin and emamectin occurs on the leaf surface. More recently, milabectin (Mesa®) has been introduced. It is a miticide with activity on piercing/sucking insects and is pending for registration.
Mode of action
Avermectins block the neurotransmitter (g-aminobutyric acid (GABA) at the neuromuscular junction in insects and mites. Visible activity, such as feeding and egg laying, stops shortly after exposure, though death may not occur for several days.
Fumigants
The fumigants are small, volatile, organic molecules that become gases at temperatures above 40°F. They are usually heavier than air and commonly contain one or more of the halogens (Cl, Br, or F). Most are highly penetrating, reaching through large masses of material. They are used to kill insects, insect eggs, nematodes, and certain microorganisms in buildings, warehouses, grain elevators, soils, and greenhouses and in packaged products such as dried fruits, beans, grain, and breakfast cereals.
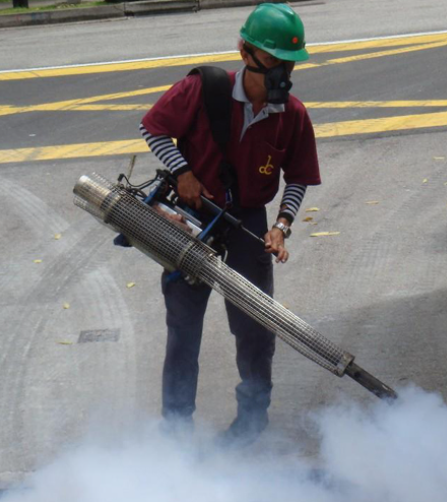
Although its use is now in decline because of environmental concerns, methyl bromide is the most heavily used of the fumigants, 68,424 metric tons worldwide in 1996, almost half of which is used in the U.S. (Aspelin & Grube 1998). The dominant use is for preplanting soil treatments, which accounted for 70% of that global total. Quarantine uses account for 5-8%, while 8% is used to treat perishable products, such as flowers and fruits, and 12% for nonperishable products, like nuts and timber. Approximately 6% is used for structural applications, as in drywood termite fumigation of infested buildings (C&E News, Nov. 9, 1998).
With the recently passed change to the Clean Air Act amendments of 1990, U.S. production and importation must be reduced 25% from 1991 levels by 1999. A 50% reduction must be achieved by 2001, followed by a 70% reduction in 2003, and a full ban of the product in 2005. Under the Montreal Protocol, developing countries have until 2015 to phase out methyl bromide production (C&E News, Nov. 9, 1998).
Some of the other common fumigants are ethylene dichloride, hydrogen cyanide, sulfuryl fluoride (Vikane®), Vapam®, Telone®II, D-D®, chlorothene, ethylene oxide, and the familiar home-use moth repellents napthalene crystals and paradichlorobenzene crystals.
Phosphine gas (PH3) has also replaced methyl bromide in a few applications, primarily for insect pests of grain and food commodities. Treatment requires the use of aluminum or magnesium phosphide pellets, which react with atmospheric moisture to produce the gas. Phosphine, however, is very damaging to fresh commodities and is highly adsorbed onto oil, thus does not perform as a soil fumigant.

Alternatives that can fully replace methyl bromide are unlikely to be available by the deadlines set for replacement. Its low cost and utility on a wide variety of pests are hard to match. Because the loss of MeBr has considerable economic consequences, EPA has made it a priority to find and register replacements. To this end some progress has been made. The chemical 1,3-dichloropropane (Telone®) was registered in 2001 for preplant soil fumigation in strawberries and tomatoes. Moreover, iodomethane (Midas®) and metam-potassium (Curtin®) are both being evaluated as soil fumigants.
Mode of action
Fumigants, as a group, are narcotics. That is, they act through means more physical than physiological. The fumigants are liposoluble (fat soluble); they have common symptomology; their effects are reversible; and their activity is altered very little by structural changes in their molecules. As narcotics, they induce narcosis, sleep, or unconsciousness, which in effect is their action on insects. Liposolubility appears to be an important factor in their action, since these narcotics lodge in lipid-containing tissues found throughout the insect body, including their nervous system.
Insect Repellents
Historically, repellents have included smoke, plants hung in dwellings or rubbed on the skin as the fresh plant or its brews, oils, pitches, tars, and various earths applied to the body. Before a more edified approach to insect olfaction and behavior was developed, it was wrongly assumed that if a substance was repugnant to humans it would likewise be repellent to annoying insects.

In recent history, the repellents have been dimethyl phthalate, Indalone®, Rutgers 612®, dibutyl phthalate, various MGK® repellents, benzyl benzoate, the military clothing repellent (N-butyl acetanilide), dimethyl carbate (Dimelone®) and diethyl toluamide (DEET, Delphene®). Of these, only DEET has survived, and is used worldwide for biting flies and mosquitoes. Most of the others have lost their registrations and are no longer available.
In 1999, EPA has registered a new insect repellent, N-methylneodecanamide. Rather than being used on humans to repel insects, it is applied to household floors and other surfaces to repel cockroaches and ants.
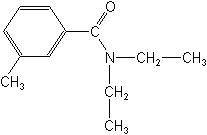
Inorganics
Inorganic insecticides are those that do not contain carbon. Usually they are white crystals in their natural state, resembling the salts. They are stable chemicals, do not evaporate, and are usually water soluble.

Sulfur, mentioned in the introduction, is very likely the oldest known, effective insecticide. Sulfur and sulfur candles were burned by our great-grandparents for every conceivable purpose, from bedbug fumigation to the cleansing of a house just removed from quarantine of smallpox. Today, sulfur is a highly useful material in integrated pest management programs where target pests specificity is important. Sulfur dusts are especially toxic to mites of every variety, such as chiggers and spider mites, and to thrips and newly-hatched scale insects. Sulfur dusts and sprays are also fungicidal, particularly against powdery mildews.
Several other inorganic compounds have been used as insecticides: mercury, boron, thallium, arsenic, antimony, selenium, and fluoride. Arsenicals have included the copper arsenate, Paris green, lead arsenate, and calcium arsenate. The arsenicals uncouple oxidative phosphorylation, inhibit certain enzymes that contain sulfhydryl (-SH) groups, and coagulate protein by causing the shape or configuration of proteins to change.
The inorganic fluorides were sodium fluoride, barium fluosilicate, sodium silicofluoride, and cryolite (Kryocide®). Cryolite has returned in recent years as a relatively safe fruit and vegetable insecticide, used in integrated pest management programs. The fluoride ion inhibits many enzymes that contain iron, calcium, and magnesium. Several of these enzymes are involved in energy production in cells, as in the case of phosphatases and phosphorylases.
Boric acid, used against cockroaches and other crawling household pests in the 1930’s and ‘40’s, has also returned. As a salt, it is non-volatile and will remain effective as long as it is kept dry and in adequate concentration. Consequently, it has the longest residual activity of any insecticide used for crawling household insects, and is quite useful in the control of all cockroach species when placed in wall voids and other protected, difficult-to-reach sites. It acts as a stomach poison and insect cuticle wax absorber.
Sodium borate (disodium octaborate tetrahydrate) (Tim-Bor®, Bora-Care®) resembles boric acid in its action. This water-soluble salt is used to treat lumber and other wood products to control decay fungi, termites, and other wood infesting pests.

The last group of inorganics is the silica gels or silica aerogels–light, white, fluffy, silicate dusts used for household insect control. The silica aerogels kill insects by absorbing waxes from the insect cuticle, permitting the continuous loss of water from the insect body, causing the insects to become desiccated and die from dehydration. These include Dri-Die®, Drianone®, and Silikil Microcel®. Drianone® is fortified with pyrethrum and synergists to enhance its effectiveness.
New Miscellaneous Insecticide Classes
Seven classes of insecticides have made their appearance in recent years. These are summarized below.
Methoxyacrylates
Fluacrypyrin (Titaron®) is an acaricide for fruit and is the only example of this class currently. It is registered for use on fruit in Japan.
FLUACRYPYRIM (Titaron®)
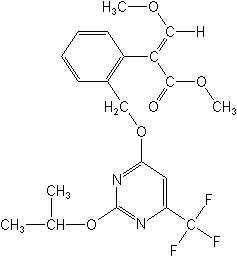
methyl (E)-2-{α-[2-isopropoxy-6-(trifluoromethyl)pyrimidin-4-yloxy]-o-tolyl}-3-methoxyacrylate
Naphthoquinones
Acequinocyl (Kanemite®, Piton®) is a miticide with insecticidal activity for pome fruit, nut crops, citrus and ornamentals. It is the only member of this group at present and the mode of action is not yet determined. It holds registrations in Korea and Japan but not in the US.
ACEQUINOCYL (Kanemite®, Piton®)
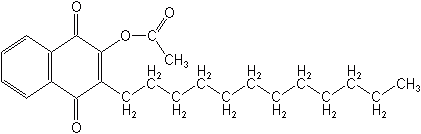
3-dodecyl-1,4-dihydro-1,4-dioxo-2-naphthyl acetate
Nereistoxin analogues
These include thiocyclam, cartap, bensultap, and thiocytap-sodium. Analogues of nereistoxin have been known for decades. They generally are stomach poisons with some contact action and often show some systemic action. A major share of the development and use of these compounds has taken place in Japan. They are based on a natural toxin of the marine worm Lumbriconereis heteropoda. Of the many analogs synthesized only those that were metabolized back to the original nereistoxin after application were active. In this sense members of this class are proinsecticides in that they are applied in their manufactured form but are known to degrade to a specific active component. The members of this group tend to be selectively active on Colopteran and Lepidopteran insect pests. Cartap (Agrotap®) is a broad spectrum insecticide with good activity against rice stem borer. Bensultap (Bancol®) is used to control the Colorado Potato beetle and other insect pests. Thiosultap-sodium (Pilarhope®) is used to control selected beetle and Lepidopteran pests on rice, vegetables and fruit trees.
Thiocyclam (Evisect®), is used for the control of similar pests in several crops. Members of this class act as acetyl choline receptor agonists at low concentrations and as channel blockers at higher concentrations. Although there has been commercial interest in thiocyclam for use in the US we do not believe there are commercial examples that are to achieve U.S. registration.
Thiocyclam (Evisect®)
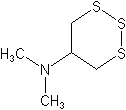
N,N-dimethyl-1,2,3-trithian-5-ylamine
Pyridine azomethin
Pymetrozine (Fulfill®), first registered in 1999 by EPA has a unique mode of action that is not fully understood. It appears to act by preventing insects from the Order Homoptera from inserting their stylus into plant tissue. Pymetrozine is used to control aphids and whiteflies in vegetables, potatoes, tobacco, deciduous citrus fruit hops and ornamentals.
PYMETROZINE (Fulfill®)
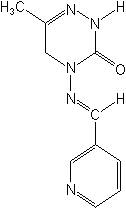
(E)-4,5-dihydro-6-methyl-4-(3-pyridylmethyleneamino)-1,2,4-triazin-3(2H)-one
Pyrimidinamines
Pyrimidifen (Miteclean®) is an insecticide and miticide. As a miticide the product controls spider and rust mites in deciduous fruits, citrus, vegetables and tea. As an insecticide it controls diamondback moth in vegetables. Very little information is available on the other member of this class, Flufenerim (S-1560), other than it is insecticidal.
PYRIMIDIFEN (Miteclean®)
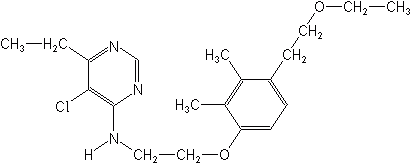
5-chloro-N-{2-[4-(2-ethoxyethyl)-2,3-dimethylphenoxy]ethyl}-6-ethylpyrimidin-4-amine
Tetronic Acids
Spirodiclofen (Envidor®) and spiromesifen (Oberon®) are the only two members of this recently introduced class. Spirodiclofen has broad-spectrum activity against mites, and controls scale crawlers and psyllad nymphs. Action is good on eggs and quiescent stages. Target crops are citrus, grapes, nuts, pome and stone fruits.
SPIRODICLOFEN (Envidor®)
3-(2,4-dichlorophenyl)-2-oxo-1-oxaspiro[4.5]dec-3-en-4-yl 2,2-dimethylbutyrate
Miscellaneous Compounds
Clofentezine (Apollo®, Acaristop®), belongs to the unique group, the tetrazines, used as an acaricide/ovicide for deciduous fruits, citrus, cotton, cucurbits, vines and ornamentals. A newer and somewhat similar agent is etoxazole (TerraSan®) which is an acaricide registered in 2002 for ornamentals grown in greenhouses. The modes of action of these two compounds are not yet understood.
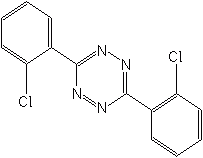
ETOXAZOLE (TerraSan®)
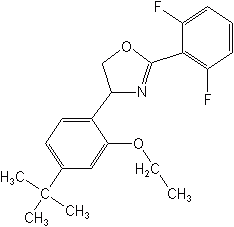
(RS)-5-tert-butyl-2-[2-(2,6-difluorophenyl)-4,5-dihydro-1,3-oxazol-4-yl]phenetole
Enzone®, sodium tetrathiocarbonate, is used only on grapes and citrus applied as a water application and irrigated into the soil. It breaks down in the soil to form carbon disulfide, which acts rapidly, decomposes quickly, and is effective against nematodes, soil insects, and soil borne diseases.
The newest agents in this category are pyridanyl and amidoflumet. Pryidalyl (S-1812) is active on Lepidoptera and thrips and has the advantage of being active against pyrethroid-resistant insects. Little more is available on amidoflumet (S-1955) other than it is an acaricide in the early stage of its development.
PYRIDALYL (S-1812)
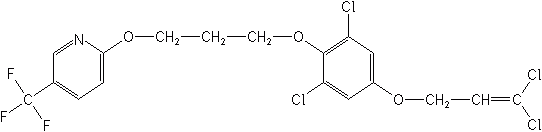
2,6-dichloro-4-(3,3-dichloroallyloxy)phenyl 3-[5-(trifluoromethyl)-2-pyridyloxy]propyl ether
AMIDOFLUMET (S-1955)
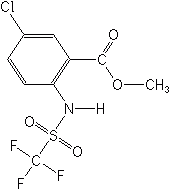
methyl 5-chloro-2-{[(trifluoromethyl)sulfonyl]amino}benzoate
Biorational Insecticides
The U.S. EPA identifies biorational pesticides as inherently different from conventional pesticides, having fundamentally different modes of action, and consequently, lower risks of adverse effects from their use. Biorational has come to mean any substance of natural origin (or man-made substances resembling those of natural origin), that has a detrimental or lethal effect on specific target pest(s), e.g., insects, weeds, plant diseases (including nematodes), and vertebrate pests, possess a unique mode of action, are non-toxic to man and his domestic plants and animals, and have little or no adverse effects on wildlife and the environment. EPA uses a similar term, biopesticides, which will be defined below.

Biorational insecticides are grouped as either (1) biochemicals (hormones, enzymes, pheromones and natural agents, such as insect and plant growth regulators), or (2) microbial (viruses, bacteria, fungi, protozoa, and nematodes). In the 1990’s the US-EPA began to emphasize a class of products known as biopesticides. EPA places biopesticides into three categories:
- Microbial pesticides (bacteria, fungi, virus or protozoa).
- Biochemicals – natural substances that control pests by non-toxic mechanisms. An example is insect pheromones.
- Plant-Incorporated protectants (PIPs) – (primarily transgenic plants, e.g., Bt corn).
EPA discloses that at the end of 2001 there were nearly 200 biopesticide active ingredients registered comprising nearly 800 products.
Characteristics that distinguish biorational and biopesticides from conventional ones include: very low orders of toxicity to non-target species, pest targets are specific, generally low use rates, rapid decomposition in the environment, usually work well in IPM programs and reduce reliance on conventional pesticide products.
The terms “biorational” and “biopesticide” overlap but are not identical. Below is an overview of what is considered as biorational insecticides. In some cases there are overlaps with botanicals (e.g., rotenone, florals, etc. and also conventional insecticides (e.g., benzoylureas). We will point out the discrepancies in classification between the biorational and biopesticide categories where they occur.
Insect Pheromones
Most insects appear to communicate by releasing molecular quantities of highly specific compounds that vaporize readily and are detected by insects of the same species. These delicate molecules are known as pheromones. The word pheromone comes from the Greek pherein, “to carry,” and hormon, “to excite or stimulate.”

Of 1,314 species of insects with confirmed attraction responses to identified pheromones, 1,260 of these pheromones are produced by females. Only 54 species use male-produced sex attractants. In a few species both sexes produce the same attractant by both sexes (Mayer & Laughlin 1990).
Pheromones are classified as either, releasers and or primers. Releasers are fast-acting and are used by insects for sexual attraction, aggregation (including trail following), dispersion, oviposition, and alarm. Primers are slow-acting and cause gradual changes in growth and development, especially in social insects by regulating caste ratios of the colony.
The five principal uses for sex pheromones are: (1) male trapping, to reduce the reproductive potential of an insect population; (2) movement studies, to determine how far and where insects move from a given point; (3) population monitoring, to determine when peak emergence or appearance occurs; (4) detection programs, to determine if a pest occurs in a limited trapping area, such as around international airports or quarantined areas; and (5) the “confusion or mating disruption” technique.
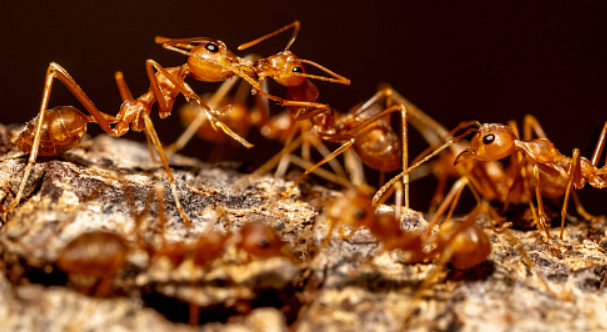
The first use of mating disruption involved gossyplure, the pink bollworm pheromone. Incorporated into small, hollow, polyvinyl fibers that permit slow release of the pheromone, it was broadcast heavily and uniformly over infested cotton fields. In mid 2002, EPA had registered 36 pheromones which comprised over 200 individual products.
Despite praise for the potential of sex pheromones, they are most practically used in survey traps to provide information about population levels, to delineate infestations, to monitor control or eradication programs, and to warn of new pest introductions.
Insect Growth Regulators
Insect growth regulators (IGRs) are chemical compounds that alter growth and development in insects. The IGRs disrupt insect growth and development in three ways: As juvenile hormones, as precocenes, and as chitin synthesis inhibitors. Juvenile hormones (JH) include ecdysone (the molting hormone), JH mimic, JH analog (JHA), and are known by their broader synonyms, juvenoids and juvegens. They disrupt immature development and emergence as adults. Precocenes interfere with the normal function of glands that produce juvenile hormones. And, chitin synthesis inhibitors, (conventional benzoylureas, buprofezin and cyromazine), affect the ability of insects to produce new exoskeletons when molting.
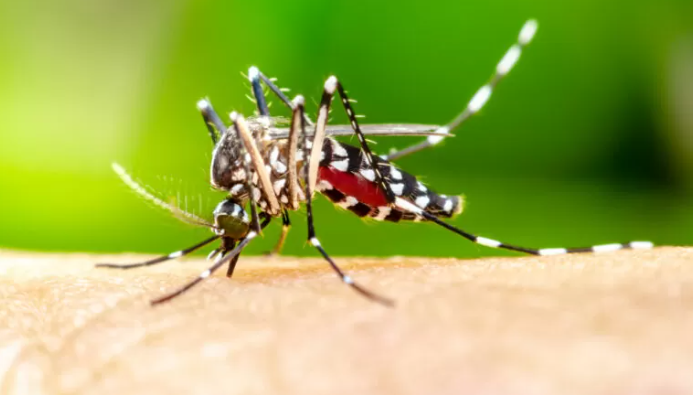
The IGRs are effective when applied in very minute quantities and generally have few or no effects on humans and wildlife. They are, however, nonspecific, since they affect not only the target species, other arthropods as well.
Instead of killing directly, IGRs interfere in the normal mechanisms of development and cause the insects to die before reaching the adult stage. One JH is the classical juvabione, found in the wood of balsam fir. Its effect was discovered quite by accident when paper towels made from this source were used to line insect-rearing containers, and the insects’ development was suppressed.
Some of these plant-derived substances actually serve to inhibit the development of insects feeding thereon, thus protecting the host plant. These are referred to broadly as antijuvenile hormones, more accurately, antiallatotropins, or precocenes. Although the mode of action of the precocenes is still unclear, it is known that they depress the level of juvenile hormone below that normally found in immature insects.
For practical purposes, IGRs are used on crops to suppress damaging insect numbers. They would be applied with the purpose of preventing pupal development or adult emergence, thus keeping the insects in the immature stages, resulting eventually in their deaths. Commercial successful pheromones have shown activity on mosquito larvae, caterpillars, and hemipterans (bugs), although effects have been observed on practically all insect orders.
Several IGRs are now registered by the EPA. The most common are: methoprene (Altosid®), a mosquito growth regulator, for use against second through fourth larval stage floodwater mosquitoes and as Precor® for indoor control of dog and cat fleas; Hydroprene (Gentrol®, Mator®) for use against all cockroaches, and stored grain pests; and kinoprene (Enstar II®), which is effective against aphids, whiteflies, mealybugs, and scales (both soft and armored) on ornamental plants and vegetable seed crops grown in greenhouses and shadehouses.
Methoprene (Altosid®)
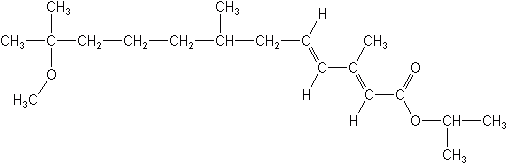
1-methylethyl (2E,4E)-11-methoxy-3,7,11-trimethyl-2,4-dodecadienoate
Fenoxycarb (Logic®, Award®, Comply®, Torus®) is a carbamate stomach insecticide that has also JH-type effects when contacted or ingested by a wide array of arthropod pests, e.g., ants, roaches, ticks, chiggers and many others. Pyriproxifen (Knack®, Esteem®, Admiral®, Archer®) is an effective molt inhibitor for a wide range of insects, but particularly useful for whitefly on cotton, citrus scales, fly-breeding sites such as livestock and poultry houses, and aquatic sites for mosquito control. Another is buprofezin (Applaud®) classed as a thiadiazine IGR. Both have given excellent results in controlling the whitefly complex, now a universal problem in U. S. cotton production.
Pyriproxifen (Knack®, Esteem®)
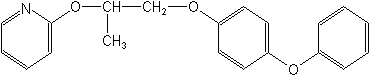
2-[1-methyl-2-(4-phenoxyphenoxy)ethoxy]pyridine
Buprofezin (Applaud®)
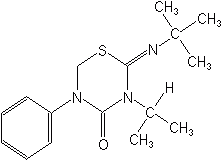
2-[(1,1-dimethylethyl)imino]tetrahydro-3-(1-methylethyl)-5-phenyl-4H-1,3,5-thiadiazin-4-one
None of the above– pyriproxifen, buprofezin, fenoxycarb or the methoprene, hydroprene group of juvenile hormone mimics are considered to be biopesticides by EPA.
Hydrazine Insecticide/IGRs
A newer class of insecticidal IGRs is the hydrazines, which includes tebufenozide, halofenozide, methoxyfenozide and chromafenozide. All are ecdysone agonists or disruptors. EPA has not classified members of this group as biopesticides. Tebufenozide (Mimic®, Confirm®), in addition to being both a stomach and contact insecticide, has also JH-IGR characteristics. It disrupts the molting process by antagonizing ecdysone, the molting hormone. Lepidopteran pests are controlled while maintaining natural populations of beneficial insect predators and parasites. Halofenozide (Mach-2®) registered in1999, is a systemic IGR, effective on cutworms, sod webworms, armyworms and white grubs, and has some ovicidal activity. It lacks the stomach or contact characteristics of tebufenozide. Methoxyfenozide (Intrepid®), like tebufenozide, is both a stomach and contact insecticide with JH-IGR qualities. It is systemic only through the roots. Pests controlled are lepidopterans such as codling moth, oriental fruit moth, European corn borer, and others. Crop candidates are cotton, corn, vegetables, pome fruit, and grapes. EPA considered methoxyfenozide as a reduced-risk candidate and first registered it in mid-2000. Chromafenozide (Matric®) is a newer member of this group, not registered in the U.S., and is used to control various lepidopteran pests in vegetables and ornamentals.
Tebufenozide (Mimic®, Confirm®)
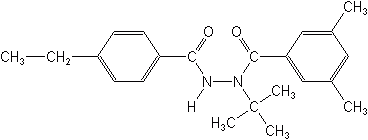
3,5-dimethylbenzoic acid 1-(1,1-dimethylethyl)-2-(4-ethylbenzoyl)hydrazide
Other Biorational Insecticides
A number of the products that we covered under botanicals and florals are also considered by many to be biorational products, and indeed, EPA includes them under the biopesticide category. Some examples include Neem oil, cinnamaldehyde, and eugenol. A new product, Virtuoso®, is a Streptomycetes-based agent that controls caterpillars but little is yet published on it, at present. Clandosan® is a naturally occurring product derived from crab and shrimp shells and used as a nematicide. It is a dried, powdered, chitin protein isolated from crustacean exoskeletons and blended with urea. It stimulates growth of beneficial soil microorganisms that control nematodes, but does not have a direct adverse effect on nematodes as such.
Microbials
Microbial insecticides obtain their name from microorganisms that are used to control certain insects. The insect disease-causing microorganisms do not harm other animals or plants. At present there are relatively few produced commercially and approved by the EPA (over 55 natural, and 16 bioengineered organisms) for use on food and feed crops. In mid-2002, the EPA list of registered microbials included 35 bacteria, 1 yeast, 17 fungi, 1 protozoan, 6 viruses, 8 bioengineered organisms and 8 transgenic crop genes.
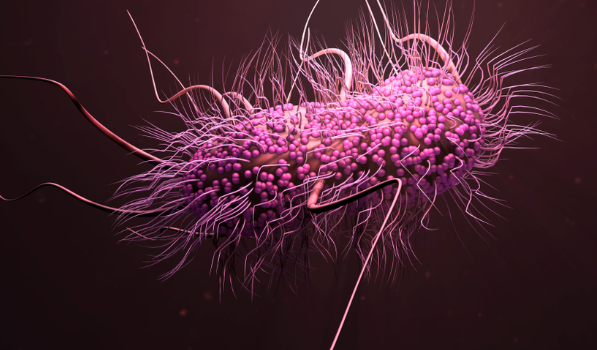
The insecticidal bacterium Bacillus thuringiensis (Bt) was discovered in the early 20th century. It occurs as a large number of subspecies that are identified among other characteristics by surface antigens, plasmid arrays, and breadth of species responding to its insecticidal action. Bt is a soil inhabiting, gram-positive sporulating bacterium that produces one or more very tiny parasporal crystals within its sporulating cells. These crystals are composed of large proteins known as delta-endotoxins. Delta-endotoxins act by binding to specific receptor sites on the gut epithelium, leading slowly to degradation of the gut lining and starvation. Thus, several days are required to kill insects that have ingested Bt products. Over time, several B. thuringiensis varieties have been discovered, each with its distinct toxicity characteristics to different insect species. B. thuringiensis var. kurstaki was the first, being the spores and crystalline delta-endotoxin as the active ingredient, and produced by B. thuringiensis Berliner, var. kurstaki, Serotype H-3a3b, HD-1, in fermentation. Products from this process control most lepidopteran pests, the caterpillars with high gut pH, which include the armyworms, cabbage looper, imported cabbage worm, gypsy moth, and spruce budworm. The next was B. thuringiensis var. israelensis, being the crystalline delta-endotoxin as the active ingredient, and produced by fermentation of B. thuringiensis Berliner, var. israelensis, Serotype H-14. These products are used primarily for the control of aquatic insects, the mosquitoes and black flies in their larval forms. Then came B. thuringiensis var. aizawai, produced by this variety, Serotype H-7, in fermentation. This product is currently registered only for the control of the wax moth larval infestations in the honey comb of honey bees. Following this came B. thuringiensis var. morrisoni, spores and delta-endotoxin produced by fermentation of Serotype 8a8b. This is again a broad spectrum Bt for most caterpillars on most crops including the home garden. B. thuringiensis var. san diego was developed for Colorado potato beetle control on all its hosts, the elm leaf beetle and other beetle larvae on a wide range of shade and ornamental trees. This was the first Bt product that was effective against coleopteran larvae. B. thuringiensis var. tenebrionis and the identical var. san diego were also developed for the Colorado potato beetle. The utilization of Bt genes transplanted into crops, which is addressed elsewhere in this document, is transforming the area of microbial pesticides.

An innovative development in the agricultural use of microbial insecticides was the addition of feeding or gustatory stimulants, making the mixtures serves as baits. The feeding stimulants attracted the caterpillars to treated foliage, which increased their consumption of the microbial. Two successfully marketed products were Coax® and Gustol®, both of which were cottonseed meal derivatives formulated as wettable powders. Both products have been discontinued.
Fungi:. Mycar® was a promising biorational miticide, a mycoacaricide, but was discontinued by the manufacturer in 1984. The microorganism was Hirsutella thompsonii, a parasitic fungus that infects and kills the citrus rust mite. Under optimum conditions H. thompsonii can infect spider mites and other nontarget mites. It was, however, consistently effective only against the citrus rust mite; thus a selective miticide.

EPA registered Metarhizium anisopliae St. F52 in mid-2002 to control various ticks, beetles, flies, gnats and thrips for non-food outdoor and greenhouse uses. Certain of the registered uses were conditional for two years pending results of tick performance studies. Another strain of this organism (St. ESF1) is also registered as a termiticide. Application was made in 1998 to register the fungus Aspergillus flavus strain AF36 as a bioinsecticide for cotton. Its purpose is to help reduce the incidence of other Aspergillus spp. that produce the highly toxic mycotoxin, aflatoxin, in cotton seed.
Protozoa: Nosema locustae is a biorational originally developed by Sandoz, Inc., in 1981, for the control of grasshoppers. Marketed under the names NOLO-Bait®;, NOLO-BB®, and Grasshopper Attack®, the microorganism is a protozoan. These have been discontinued although the registrations remain.

Nematodes: There were two commercial nematode products available for termite control, Spear® and Saf T-Shield®. The nematode, Neoaplectana carpocapsae, in the family Steirnernamatidae, is specific for subterranean termites. It kills all stages of these termites by delivering a pathogenic bacterium, Xenorhabdus spp., which is lethal within 48 hours after penetration. Unfortunately, neither product succeeded commercially.
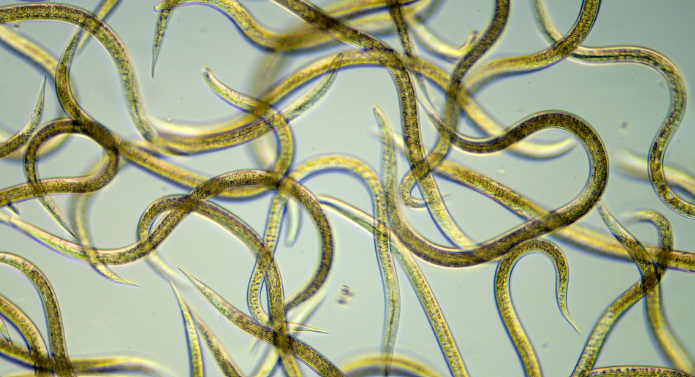
A New Horizon: Transgenic Pesticides
The concept that organisms and crops could be engineered to augment pest control was well known in the 1970’s. By the mid 1980s, one company, Monsanto had committed to a research program designed to create crop protection products through the application of biotechnology. Charles (2001) has produced a very readable history of pesticide-related transgenic crops and this book is recommended to those who want to understand how this new technology unfolded.

Transgenic organisms are genetically altered by artificial introduction of DNA from another organism. The artificial gene sequence is referred to as a transgene. Plants with such transgenes are also referred to as being genetically modified (GM). Plants that emulate insecticides are those altered to induce em>insect-resistance (also called plant pesticides or plant incorporated protectants0. The purpose of the following paragraphs is to summarize what biotechnology has contributed to insecticide science in the course of just the last decade or so.
Research built on the elucidation of the genetic code in the early 1950’s and culminating in the 1990’s allowed those using the techniques of biotechnology to move genes coding for specific traits from selected organism to crop cells. Such altered cells were then regenerated to viable crop plants through tissue culture. Several transgenic crops have thus been and are being created from backcrossing the selected traits into elite seed lines. The result has led us to plant pesticides.

Plant pesticides are defined by EPA as plants that have been genetically engineered to contain the delta-endotoxin genes from Bacillus thuringiensis. This definition will expand as genes from additional sources are incorporated into plants.
In 1995, EPA registered the first plant pesticide. It was Monsanto’s Bt-cotton containing B. t. Cry1Ac delta-endotoxin, following more than a decade of research. This novel form of cotton was introduced experimentally in 1995 as Bollgard® cotton, resistant to tobacco budworm, cotton bollworm, and pink bollworm with activity on other minor lepidopteran pests. Bt-enhanced cotton, corn and other insect resistant crops produce one or more crystalline proteins that disrupt the gut lining of susceptible insect pests feeding on their tissues which cause the pests to stop feeding and die. Several plant pesticides have been introduced in the U.S. since 1995. Some of these have been very successful commercially while others, such as, NewLeaf® Potatoes, have been withdrawn from the market.
In some subsequent product introductions the performance of these plant pesticides have been enhanced or augmented by use of stacked genes. This means that more than one transgene is introduced into the same crop to achieve multiple desired characteristics.
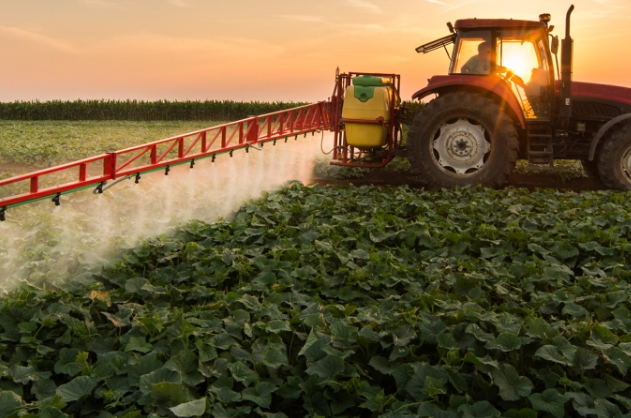
In the U.S. the proportion of total planted acres of B.t. corn, grew from 18% in 2000 to 26 % in 2003, and this does not include stacked-gene plant insecticides. The proportions for cotton were even higher if stacked-gene varieties were included.
Three U.S. federal agencies (USDA, FDA and EPA) regulate the release and use of transgenic plants and plant pesticides under a coordinated framework. There is a sharing and partitioning of biotechnology regulatory responsibility among these three agencies. The approach of each agency is similar, in that, they each evaluate risks from a sound science perspective and regulate individual products on a case-by-case basis. There is considerable reliance on comparing transgenic organisms with their conventional counterparts that have a known history of safe use. The emphasis during the regulatory review is to assure that the GM organism will not produce harmful toxins, allergens, etc., and will not, once released, cause adverse effects (such as become pests themselves).
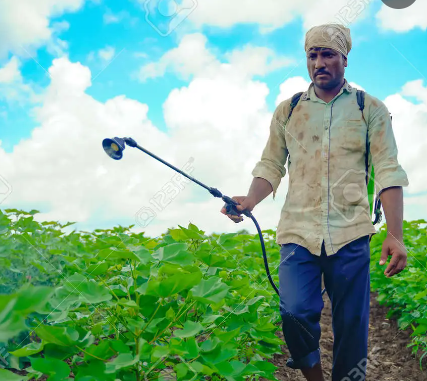
The first significant biotech crop introductions, including plant insecticides, occurred in 1995, but commercial introductions are advancing rapidly. A Reuters article dated Jan. 15, 2003, cited on the ISAAA website (http://www.isaaa.org/) projects global transgenic sales (including plant insecticides and herbicide tolerance) in excess of $4 billion in 2003, while Phillips McDougall, the authoritative consultant and publisher projects such sales in 2006 to be more than $5 billion in 2006.
Pesticides
Pesticides are substances that are meant to control pests. This includes herbicide, insecticide, nematicide, molluscicide, piscicide, avicide, rodenticide, bactericide, insect repellent, animal repellent, microbicide, fungicide, and lampricide. The most common of these are herbicides which account for approximately 80% of all pesticide use. Most pesticides are intended to serve as plant protection products (also known as crop protection products), which in general, protect plants from weeds, fungi, or insects. As an example, the fungus Alternaria solani is used to combat the aquatic weed Salvinia.
In general, a pesticide is a chemical (such as carbamate) or biological agent (such as a virus, bacterium, or fungus) that deters, incapacitates, kills, or otherwise discourages pests. Target pests can include insects, plant pathogens, weeds, molluscs, birds, mammals, fish, nematodes (roundworms), and microbes that destroy property, cause nuisance, or spread disease, or are disease vectors. Along with these benefits, pesticides also have drawbacks, such as potential toxicity to humans and other species.

There are many different types of pesticides, each is meant to be effective against specific pests. The term “-cide” comes from the Latin word “to kill.”
Algaecides are used for killing and/or slowing the growth of algae.
Antimicrobials control germs and microbes such as bacteria and viruses.
Biopesticides are made of living things, come from living things, or they are found in nature.
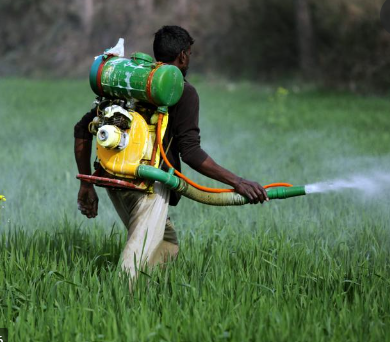
Desiccants are used to dry up living plant tissues.
Defoliants cause plants to drop their leaves.
Disinfectants control germs and microbes such as bacteria and viruses.
Foggers (total release foggers) are used to kill insects that are in the open and touch the pesticides.
Fungicides are used to control fungal problems like molds, mildew, and rust.
Herbicides kill or inhibit the growth of unwanted plants, aka weeds.
Illegal and Counterfeit Pesticides are imported or sold illegally.
Insecticides are used to control insects.
Insect Growth Regulators disrupt the growth and reproduction of insects.
Minimum Risk Pesticides are exempt from EPA registration, but many states require them to be registered.

Miticides control mites that feed on plants and animals. Mites are not insects, exactly.
Molluscicides are designed to control slugs, snails and other molluscs.
Mothballs are insecticides used to kill fabric pests by fumigation in sealed containers.
Natural and Biological Pesticides control pests using things found in nature, or manmade versions of things found in nature.
Organic Pesticides are usually not manmade and tend to have natural substances like soaps, lime sulfur, and hydrogen peroxide as ingredients.
Ovicides are used to control eggs of insects and mites.
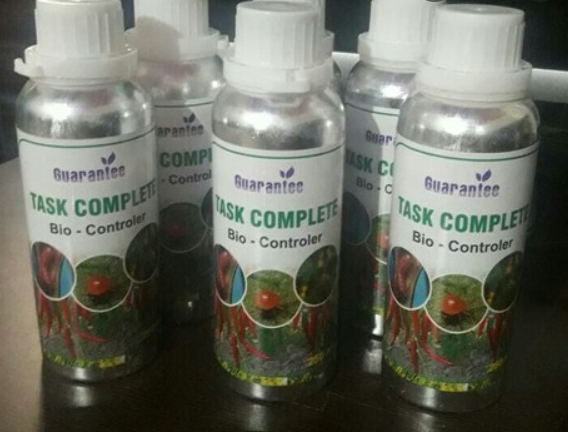
Pheromones are biologically active chemicals used to attract insects or disrupt their mating behavior. The ratio of chemicals in the mixture is often species-specific.
Plant Growth Regulators are used to alter the growth of plants. For example, they may induce or delay flowering.
Repellents are designed to repel unwanted pests, often by taste or smell.
Rodenticides are used to kills rodents like mice, rats, and gophers.
Synergists make certain pesticides more effective, but they are not effective when used alone.
Treated Seeds are coated with a pesticide to limit crop damage from fungus and insects.
Wood Preservatives are used to make wood resistant to insects, fungus and other pests.
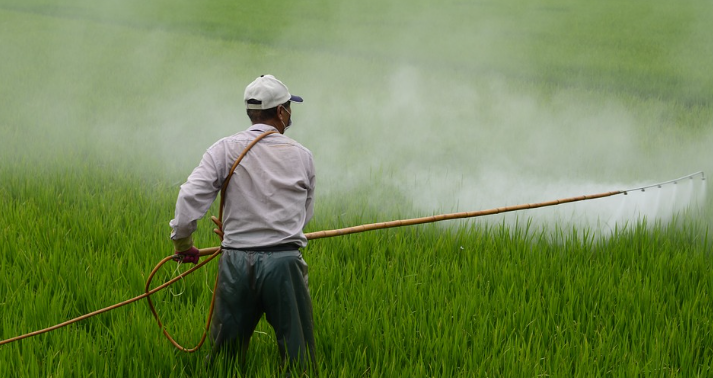
Active Ingredients
Active ingredients are the chemicals in a pesticide product that act to control the pests. Active ingredients must be identified by name on the pesticide product’s label together with its percentage by weight.
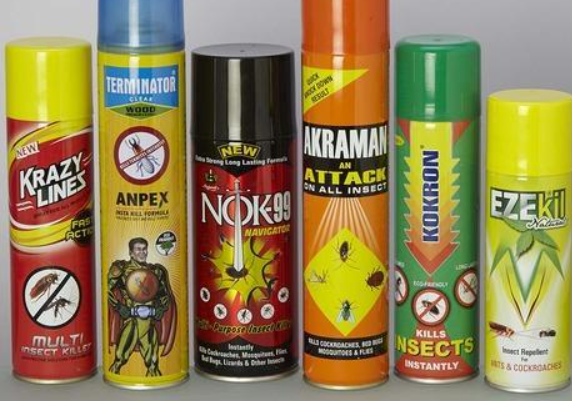
There are several categories of active ingredients:
- Conventional, which are all ingredients other than biological pesticides and antimicrobial pesticides.
- Antimicrobial, which are substances or mixtures of substances used to destroy or suppress the growth of harmful microorganisms whether bacteria, viruses, or fungi on inanimate objects and surfaces.
- Biopesticides, which are types of ingredients derived from certain natural materials.
Inert Ingredients
Pesticide products contain at least one active ingredient and other intentionally added inert ingredients. Called “inert ingredients” by the federal law, they are combined with active ingredients to make a pesticide product. Inerts are chemicals, compounds, and other substances, including common food commodities (e.g., certain edible oils, spices, herbs) and some natural materials (e.g., beeswax, cellulose).
The name “inert” does not mean non-toxic. All inert ingredients must be approved by EPA before they can be included in a pesticide. We review safety information about each inert ingredient before approval. If the pesticide will be applied to food or animal feed, a food tolerance is required for each inert ingredient in the product, and we may limit the amount of each inert ingredient in the product.
Inert ingredients play key roles in pesticide effectiveness and product performance. Examples of functions inerts can serve include:
- Act as a solvent to help the active ingredient penetrate a plant’s leaf surface.
- Improve the ease of application by preventing caking or foaming.
- Extend the product’s shelf-life.
- Improve safety for the applicator.
- Protect the pesticide from degradation due to exposure to sunlight.

Under federal law, the identity of inert ingredients is confidential business information. The law does not require manufacturers to identify inert ingredients by name or percentage on product labels. In general, only the total percentage of all inert ingredients is required to be on the pesticide product label.Information on inert ingredients: Inert Ingredients Overview and GuidanceInert Ingredient RegulationOther/Inert Ingredients in PesticidesEXITEXIT EPA WEBSITE
Evaluating Pesticide Ingredients
Before manufacturers can sell pesticides in the United States, EPA must evaluate them thoroughly to ensure that they meet federal safety standards to protect human health and the environment. We grant a “registration” or license that permits a pesticide’s distribution, sale, and use only after the company meets the scientific and regulatory requirements.

In evaluating a pesticide registration application, we assess a wide variety of potential human health and environmental effects associated with use of the pesticide. Potential registrants must generate scientific data necessary to address concerns pertaining to the:
- identity,
- composition,
- potential adverse effects, and
- environmental fate
of each pesticide.
Chemical fertilizers
A fertilizer (American English) or fertiliser (British English; see spelling differences) is any material of natural or synthetic origin that is applied to soil or to plant tissues to supply plant nutrients. Fertilizers may be distinct from liming materials or other non-nutrient soil amendments. Many sources of fertilizer exist, both natural and industrially produced.[1] For most modern agricultural practices, fertilization focuses on three main macro nutrients: Nitrogen (N), Phosphorus (P), and Potassium (K) with occasional addition of supplements like rock dust for micronutrients. Farmers applying these fertilizers in a variety of ways: through dry or pelletized or liquid application processes, using large agricultural equipment or hand-tool methods.
Historically fertilization came from natural or organic sources: compost, animal manure, human manure, harvested minerals, crop rotations and byproducts of human-nature industries (i.e. fish processing waste, or bloodmeal from animal slaughter). However, starting in the 19th century, after innovations in plant nutrition, an agricultural industry developed around synthetically created fertilizers. This transition was important in transforming the global food system, allowing for larger-scale industrial agriculture with large crop yields. In particular, nitrogen-fixing chemical processes such as the Haber process at the beginning of the 20th century, amplified by production capacity created during World War II led to a boom in using nitrogen fertilizers. In the latter half of the 20th century, increased use of nitrogen fertilizers (800% increase between 1961 and 2019) have been a crucial component of the increased productivity of conventional food systems (more than 30% per capita) as part of the so-called “Green Revolution“.
Fertilizers are classified in several ways. They are classified according to whether they provide a single nutrient (e.g., K, P, or N), in which case they are classified as “straight fertilizers.” “Multinutrient fertilizers” (or “complex fertilizers”) provide two or more nutrients, for example N and P. Fertilizers are also sometimes classified as inorganic (the topic of most of this article) versus organic. Inorganic fertilizers exclude carbon-containing materials except ureas. Organic fertilizers are usually (recycled) plant- or animal-derived matter. Inorganic are sometimes called synthetic fertilizers since various chemical treatments are required for their manufacture.
Single nutrient (“straight”) fertilizers
The main nitrogen-based straight fertilizer is ammonia or its solutions. Ammonium nitrate (NH4NO3) is also widely used. Urea is another popular source of nitrogen, having the advantage that it is solid and non-explosive, unlike ammonia and ammonium nitrate, respectively. A few percent of the nitrogen fertilizer market (4% in 2007) has been met by calcium ammonium nitrate (Ca(NO3)2 · NH4 · 10 H2O).
The main straight phosphate fertilizers are the superphosphates. “Single superphosphate” (SSP) consists of 14–18% P2O5, again in the form of Ca(H2PO4)2, but also phosphogypsum (CaSO4 · 2 H2O). Triple superphosphate (TSP) typically consists of 44–48% of P2O5 and no gypsum. A mixture of single superphosphate and triple superphosphate is called double superphosphate. More than 90% of a typical superphosphate fertilizer is water-soluble.
The main potassium-based straight fertilizer is muriate of potash (MOP). Muriate of potash consists of 95–99% KCl, and is typically available as 0-0-60 or 0-0-62 fertilizer.
Multinutrient fertilizers
These fertilizers are common. They consist of two or more nutrient components.Binary (NP, NK, PK) fertilizers

Major two-component fertilizers provide both nitrogen and phosphorus to the plants. These are called NP fertilizers. The main NP fertilizers are monoammonium phosphate (MAP) and diammonium phosphate (DAP). The active ingredient in MAP is NH4H2PO4. The active ingredient in DAP is (NH4)2HPO4. About 85% of MAP and DAP fertilizers are soluble in water.NPK fertilizersMain article: Labeling of fertilizer
NPK fertilizers are three-component fertilizers providing nitrogen, phosphorus, and potassium. There exist two types of NPK fertilizers: compound and blends. Compound NPK fertilizers contain chemically bound ingredients, while blended NPK fertilizers are physical mixtures of single nutrient components.

NPK rating is a rating system describing the amount of nitrogen, phosphorus, and potassium in a fertilizer. NPK ratings consist of three numbers separated by dashes (e.g., 10-10-10 or 16-4-8) describing the chemical content of fertilizers.[22][23] The first number represents the percentage of nitrogen in the product; the second number, P2O5; the third, K2O. Fertilizers do not actually contain P2O5 or K2O, but the system is a conventional shorthand for the amount of the phosphorus (P) or potassium (K) in a fertilizer. A 50-pound (23 kg) bag of fertilizer labeled 16-4-8 contains 8 lb (3.6 kg) of nitrogen (16% of the 50 pounds), an amount of phosphorus equivalent to that in 2 pounds of P2O5 (4% of 50 pounds), and 4 pounds of K2O (8% of 50 pounds). Most fertilizers are labeled according to this N-P-K convention, although Australian convention, following an N-P-K-S system, adds a fourth number for sulfur, and uses elemental values for all values including P and K.
Micronutrients
Micronutrients are consumed in smaller quantities and are present in plant tissue on the order of parts-per-million (ppm), ranging from 0.15 to 400 ppm or less than 0.04% dry matter. These elements are often required for enzymes essential to the plant’s metabolism. Because these elements enable catalysts (enzymes), their impact far exceeds their weight percentage. Typical micronutrients are boron, zinc, molybdenum, iron, and manganese. These elements are provided as water-soluble salts. Iron presents special problems because it converts to insoluble (bio-unavailable) compounds at moderate soil pH and phosphate concentrations. For this reason, iron is often administered as a chelate complex, e.g., the EDTA or EDDHA derivatives. The micronutrient needs depend on the plant and the environment. For example, sugar beets appear to require boron, and legumes require cobalt while environmental conditions such as heat or drought make boron less available for plants.

“Organic fertilizers” can describe those fertilizers with an organic – biologic – origin—that is, fertilizers derived from living or formerly living materials. Organic fertilizers can also describe commercially available and frequently packaged products that strive to follow the expectations and restrictions adopted by “organic agriculture” and ”environmentally friendly” gardening – related systems of food and plant production that significantly limit or strictly avoid the use of synthetic fertilizers and pesticides. The “organic fertilizer” products typically contain both some organic materials as well as acceptable additives such as nutritive rock powders, ground sea shells (crab, oyster, etc.), other prepared products such as seed meal or kelp, and cultivated microorganisms and derivatives.
Fertilizers of an organic origin (the first definition) include animal wastes, plant wastes from agriculture, seaweed, compost, and treated sewage sludge (biosolids). Beyond manures, animal sources can include products from the slaughter of animals – bloodmeal, bone meal, feather meal, hides, hoofs, and horns all are typical components.Organically derived materials available to industry such as sewage sludge may not be acceptable components of organic farming and gardening, because of factors ranging from residual contaminants to public perception. On the other hand, marketed “organic fertilizers” may include, and promote, processed organics because the materials have consumer appeal. No matter the definition nor composition, most of these products contain less-concentrated nutrients, and the nutrients are not as easily quantified. They can offer soil-building advantages as well as be appealing to those who are trying to farm / garden more “naturally”.
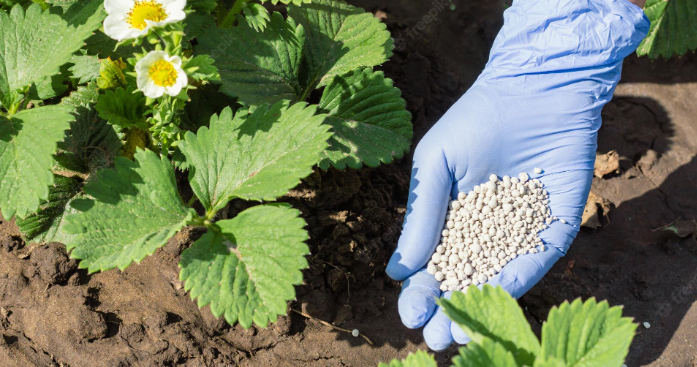
In terms of volume, peat is the most widely used packaged organic soil amendment. It is an immature form of coal and improves the soil by aeration and absorbing water but confers no nutritional value to the plants. It is therefore not a fertilizer as defined in the beginning of the article, but rather an amendment. Coir, (derived from coconut husks), bark, and sawdust when added to soil all act similarly (but not identically) to peat and are also considered organic soil amendments – or texturizers – because of their limited nutritive inputs. Some organic additives can have a reverse effect on nutrients – fresh sawdust can consume soil nutrients as it breaks down, and may lower soil pH – but these same organic texturizers (as well as compost, etc.) may increase the availability of nutrients through improved cation exchange, or through increased growth of microorganisms that in turn increase availability of certain plant nutrients. Organic fertilizers such as composts and manures may be distributed locally without going into industry production, making actual consumption more difficult to quantify.

What Is Chemical Fertilizer?
The term “chemical fertilizer” refers to any number of synthetic compound substances created specifically to increase crop yield. Some chemical fertilizers, for example, are “nitrogenous” — containing nitrogen — while others are phosphate-based. Other fertilizers are potassium.
Complex (or blended) chemical fertilizers often contain a mix of ammonium phosphate, nitrophosphate, potassium, and other nutrients.
As you learn about the importance of fertilizer, it’s important to note that the right chemical fertilizer for you depends on the climate and the type of crop you’re growing — check with a Fruit Growers Supply Company in your area for more information.
The Importance of Using Chemical Fertilizers
When it comes to the advantages of using fertilizers in agriculture, it’s all about efficiency. Chemical fertilizers allow growers to maximize their crop yield on a specific piece of land — the more the plant grows, the better. Fertilizer works to ensure that each piece of land produces as efficiently as possible.
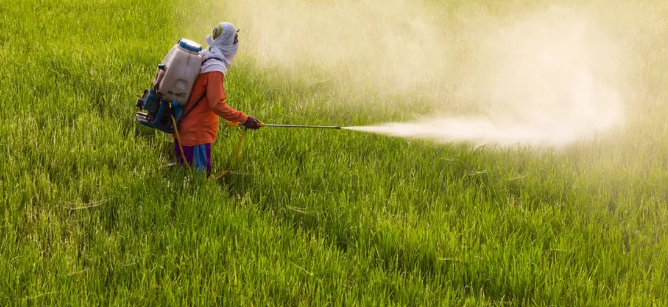
As long as you know how to approach the use of chemical fertilizers, they can even make otherwise poor quality land produce significant yields.
How Can Different Fertilizer Brands Vary?
Now that you understand the importance of fertilizer, the next step is choosing the right fertilizer for your unique requirements. You might be a bit overwhelmed when it comes to the many different brands operating in the fertilizer space. That said, don’t worry — it’s usually fairly easy to narrow down your selection, depending on your specific needs.

First, you need to determine how you intend to use your fertilizer. For example, someone interested in a ‘homeowner blend’ (something used for lawns, gardens, and so on) would likely benefit from a fertilizer brand that specializes in high-filler fertilizer blends.
A fertilizer high in filler contains less active chemical fertilizer than industrial blends, making it a perfect option for anyone looking to keep their lawn looking top-notch. These consumer blends typically incorporate low, slow-release nutrients that allow for continued growth over time.
In other words, the importance of fertilizer depends largely on context. Make sure you choose a brand and type that aligns with your specific goals, or you might not see ideal results.
Likewise, you may consider an organic fertilizer. While organic fertilizers have their time and place, chemical fertilizer is often faster, more efficient, and much more affordable than the organic alternative.

On the other hand, a homeowner fertilizer blend is certainly not ideal for a bigger operation where crop yield and sustainability are important. Commercial chemical fertilizers service large-scale areas and are tailor-made for specific crops and climates.
Why Are Fertilizers Used? What Types of Advantages Do They Offer?
Fertilizers maximize crop yield and increase plant production. Used correctly, chemical fertilizers can dramatically increase yield and turn otherwise poor soil into productive land. As you might imagine, the importance of fertilizer scales with the size of your growing operation.

And the Advantages?
Chemical fertilizers are designed specifically to support particular plants. Improvement can be recognized almost immediately — the plants absorb the nutrients quickly and the results are quickly visible.
On top of that, chemical fertilizers provide exactly what plants need to grow with minimal filler, and they’re highly regulated. Best of all, chemical fertilizers are incredibly cost-effective.

Are There Any Downsides?
Used correctly, chemical fertilizers are a brilliant and cost-effective way to produce high yields. However, as you consider the importance of fertilizer, make sure you don’t accidentally use too much of a good thing.
Overuse of chemical fertilizers can degrade the soil — it’s important to take the proper steps to restore the soil’s natural structure and balance. Overuse of chemical fertilizers may also disrupt the acidity of the soil.
Bibliography
- https://en.wikipedia.org/wiki/Insecticide
- https://ipmworld.umn.edu/ware-intro-insecticides
- https://en.wikipedia.org/wiki/Pesticide
- http://npic.orst.edu/ingred/ptype/index.html
- https://www.epa.gov/ingredients-used-pesticide-products/basic-information-about-pesticide-ingredients
- https://en.wikipedia.org/wiki/Fertilizer
Acknowledgment:
The success and final outcome of this project required a lot of guidance and assistance from many people and I am extremely privileged to have got this all along the completion of my project. All that I have done is only due to such supervision and assistance and I would not forget to thank them.
I respect and thank Mr./Ms. [NAME 1], for providing me an opportunity to do the project work in [VENUE] and giving us all support and guidance which made me complete the project duly. I am extremely thankful to [her/him] for providing such a nice support and guidance, although he had busy schedule managing the corporate affairs.
I owe my deep gratitude to our project guide [NAME 2], who took keen interest on our project work and guided us all along, till the completion of our project work by providing all the necessary information for developing a good system.
I would not forget to remember [NAME 3 AND NAME 4], of [COMPANY NAME] for their encouragement and more over for their timely support and guidance till the completion of our project work.
I heartily thank our internal project guide, [Name 5], [Position] , [Department] for her/his guidance and suggestions during this project work.
I am thankful to and fortunate enough to get constant encouragement, support and guidance from all Teaching staffs of [Department name] which helped us in successfully completing our project work. Also, I would like to extend our sincere esteems to all staff in laboratory for their timely support.